Manuel Edday Farfán-Beltrán a, Omar Arellano-Aguilar b, Alex Córdoba-Aguilar a, *
a Universidad Nacional Autónoma de México, Instituto de Ecología, Departamento de Ecología Evolutiva, Apartado postal 70-275, Ciudad Universitaria, 04510 Ciudad de México, Mexico
b Universidad Nacional Autónoma de México, Facultad de Ciencias, Departamento de Ecología y Recursos Naturales, Circuito Interior s/n, Ciudad Universitaria, 04510 Ciudad de México, Mexico
*Corresponding author: acordoba@iecologia.unam.mx (A. Córdoba-Aguilar)
Received: 11 November 2021; accepted: 28 March 2023
Abstract
Many urban environments are places with strong stressors that substantially modify water quality. Although tests evaluating water quality are usually physico-chemical, including biological components can also provide relevant information. Lake Chalco, at the border between Mexico City and Estado de México, interacts with the surrounding human population. We investigated some water quality parameters and the community of macroinvertebrates living in Lake Chalco. From January to October 2017, we sampled water along the shoreline in 4 permanent areas of the lake. We recorded the NH4+, NO3-N, NO2-N, total P, Zn, Cu2+, ORP, DO, TDS, conductivity, and pH. In addition, we characterized the richness, composition, and abundance of the macroinvertebrate community. There was spatial and temporal variation in physico-chemical parameters, perhaps due to the agricultural activity around the lake. Nevertheless, water quality was unable to be placed in a category of the national law. Four out of 20 macroinvertebrate RTU’s significantly correlated with environmental variables. Thus, no bioindicators could be proposed. In general, lake water quality is poor, so it is not recommended for anthropic activities. Yet, the lake may be important as a center of dispersion for aquatic invertebrates.
Keywords: Urbanization; Tláhuac; Pollution; Macroinvertebrates
© 2023 Universidad Nacional Autónoma de México, Instituto de Biología. This is an open access article under the CC BY-NC-ND license
(http://creativecommons.org/licenses/by-nc-nd/4.0/).
Entendiendo el impacto de los parámetros físico-químicos en los invertebrados acuáticos en el lago de Chalco, Ciudad de México
Resumen
Muchos ecosistemas urbanos son sitios con estresores fuertes que modifican sustancialmente la calidad del agua. Aunque las pruebas para evaluar la calidad del agua suelen ser físico-químicas, el incluir componentes biológicos puede proporcionar información relevante. El lago de Chalco, que se encuentra en la frontera entre la Ciudad de México y el Estado de México, interactúa con la población humana circundante. En este trabajo, investigamos algunos parámetros de calidad del agua y la comunidad de macroinvertebrados que viven en él. De enero a octubre del 2017, muestreamos alrededor de la orilla del lago en 4 áreas. Registramos la concentración de NH4+, NO3-N, NO2-N, P total, Zn, Cu2+, POR, OD, SDT, conductividad y pH. Adicionalmente, caracterizamos la riqueza, composición y abundancia de la comunidad de macroinvertebrados. Existió variación temporal y espacial en los parámetros físico-químicos, probablemente debido a la actividad agrícola alrededor del lago. Sin embargo, la calidad del agua no pudo ser colocada en alguna de las 4 categorías que establece la ley nacional. Cuatro de 20 RTU’s de macroinvertebrados estuvieron significativamente correlacionados con las variables ambientales, pero no se puede sugerir su uso como bioindicadores. En general, la calidad del agua es mala para actividades humanas. Sin embargo, el lago aún podría actuar como un centro de dispersión.
Palabras clave: Urbanización; Tláhuac; Contaminación; Macroinvertebrados
© 2023 Universidad Nacional Autónoma de México, Instituto de Biología. Este es un artículo Open Access bajo la licencia CC BY-NC-ND
Introduction
Many urban water bodies are under strong pressure from land use change, overexploitation, and constant influx of contaminants. As a result, these environments can present eutrophication, the presence of exotic species, and general trophic fragmentation that puts ecosystem integrity at risk (McDonald et al., 2013; Monteiro-Junior et al., 2014a; Villalobos-Jiménez et al., 2016). One of the aquatic components most affected by urbanization is the biota (Knop, 2016). The effect of urbanization on the biota is known to depend on both the magnitude of the pressures and on organisms’ intrinsic traits (Monteiro-Júnior et al., 2014b). However, the general trend is that diversity decreases as urbanization increases. This process has been shown to occur both over time (Kozlowski & Bondallaz, 2013) and through space (Jeanmougin et al., 2014; Monteiro-Junior et al., 2014b; Samways & Steytler, 1996).
Water quality was defined as “a measure of the condition of water relative to the requirements of 1 or more species and/or any human need or purpose” (Johnson et al., 1997). According to this definition, the practical interpretation depends directly on the intended use of the resource. For example, in Mexico, the document that characterizes water quality is the Federal Law of National Water Rights (Conagua, 2019). This law considers 4 categories: source for public urban use, agricultural irrigation, protection for freshwater life, and protection for coastal and estuarine waters.
While water quality evaluations are traditionally based on physico-chemical analyses, it is desirable to complement that information with biological monitoring (Norris & Morris, 1995). This is not trivial, since it implies a recognition that the biota and its interactions are affected by human factors and management, with some organisms responding more quickly than others (Paoletti, 1999). In this way, if some physiological processes, species, or communities are significantly negatively associated with some attribute of interest in the ecosystem, they can be used to generate bioindicators (Holt & Miller, 2010). Among the different biological groups, macroinvertebrates have been widely used in studies of water quality due to their high abundance, well-known tolerance to pollutants, restricted mobility, range of life cycle strategies and feeding habits, and complete dependence on the conditions in the sites they inhabit (Oleson, 2013). Given the practical advantages of bioindicators, some international entities, such as the World Health Organization (WHO; Bartram et al., 1996) or local agencies, such as the government of the State of Maine in the USA (Department of Environmental Protection, 2014) have developed manuals on the use of living organisms to design monitoring programs. Despite these actions, the degree of knowledge of their effectiveness is still variable among regions (Oertli, 2008). In addition, assigning taxonomic groups above the genus level into a single tolerance category can lead to error, since in some cases there is variation (Resh & Unzicker, 1975). Given these considerations, it is important to characterize the biota at each site where the bioindicator is intended to be used, as well as their specific tolerance to the parameters evaluated.
Lake Chalco, also known as Lake Tláhuac-Xico, is located on the periphery of Mexico City; it originated from subsidence due to overexploitation of groundwater (Ortiz & Ortega, 2007). Despite its current extent and the amount of water stored, there are few studies characterizing its water quality (Robles-Palacios, 2018), much less its aquatic biodiversity. Due to its recent origin, the lake sits on communal lands for traditional agriculture and small livestock purposes, so its access is restricted to landowners (called “ejidatarios”).
Given that the lake has decreased in surface area for these activities, many “ejidatarios” have reduced their income (only those with land properties located off the lake periphery are able to work). To date, there is no use of the water for irrigation or livestock. On the other hand, it is considered that the lake currently meets the different criteria to be considered a RAMSAR site: a) a site of cultural importance given its prehispanic history (Alcocer & Bernal-Brooks, 2010); b) the occurrence of 4 threatened species according to national legislation; and c) an important nesting site for birds (e.g., more than 25,000 individuals during the winter season [Ayala-Pérez et al., 2013]). There are biologically relevant classifications related to water quality, such as those concerned with eutrophication. Nevertheless, given that “ejidatarios” are currently planing to promote the establishment of a natural reserve, information regarding water quality in terms of national laws, and the relationship of water quality with macroinvertebrates may be useful for long term monitoring and management.
Our objectives in this study were: a) to assess 11 physico-chemical parameters in Lake Chalco related to water quality to determine whether it meets some of the criteria established by the national law; b) to record the macroinvertebrates present to the finest taxonomical level possible; and c) to determine whether there are significant relationships between relevant recognizable taxonomic units (RTU’s) present and physico-chemical parameters that allow us to propose some species as bioindicators.
Materials and methods
Lake Chalco is a recently formed body of water (ca. 1985) in the eastern portion of the Mexican Basin, between 19°14’56” – 19°18’31” N, 98°57’33” – 98°59’36” W (Ortiz & Ortega, 2007). Although it is located in the same place as one of the largest lakes in the country during prehispanic times, that lake was completely dried by the beginning of the last century due to agricultural use (Servín, 2005). In 1984, a system of 14 wells was created (Sedesol, 2011), which compacted the soil of the lake bed at a rate of 40 cm per year. This generated an impermeable depression that grew in area and depth. Currently, it is estimated that the depression has surpassed 15 m at its deepest point and occupies an area over 1,000 ha (Ortiz & Ortega, 2007). The lake has clayey and generally salinated soil (Domínguez-Rubio, 1997), with vegetation dominated by Typha latifolia. Given that the line of wells that formed the lake continue operations, and that there is a perpendicular highway that crosses the depression, the lake is divided into several sections, which were considered in the analyses (Fig. 1).
In order to capture some of the variability of the physico-chemical parameters and richness and diversity of macroinvertebrates of the lake, we visited it 4 times over the course of 1 year (January, April, July, and October 2017, which correspond to the winter, spring, summer, and fall seasons, respectively). The distribution of sampling sites was determined systematically every 300 m along the shoreline, following the recommendation of DiFranco (2014) to avoid sampling macroinvertebrates at depths greater than 1 m. This distance was chosen because it lies where “ejidatarios” come into contact with the lake. Sites that were not reachable due to roadways or artificial canals were excluded (Fig. 1). This resulted in a total of 30 sampling sites.
At each of the sites, we took 3 water samples 1 m from the shoreline and separated from each other by 1 m following a straight line, 5 cm above the sediment. This was done between 10:00 and 15:00 h. These samples were stored in 120 mL capacity high density polyethylene containers and were immediately placed into insulated containers with crushed ice. Each sample was filtered without being removed from the ice with Whatman® number 4 filter paper to remove particles greater than 25 µm. Then, they were stored in the dark at 4 °C until analysis in the laboratory within 10 days of sampling at the Unidad de Análisis Ambiental, Facultad de Ciencias-UNAM. Ammonia (NH4+), nitrite (NO3-N), nitrate (NO2-N), total phosphorus (P), zinc (Zn), and copper (Cu2+) were quantified using a multiparameter spectrophotometer (HI83099, Hanna Instruments®) using specific reagents for each test following the manufacturer’s instructions.
For sampling physico-chemical variables, we used a multiparameter reader (model HI9829, Hanna Instruments®) with a HI7609829-2 probe to measure DO; HI7609829-0 for pH, and HI7609829-3 for Total Dissolved Solids (TDS) and Oxidation/Reduction Potential (ORP). At each site, we took 3 measurements in the same place where the water samples were collected and averaged these values. These measurements were stored in the internal memory of the multiparameter reader and later transferred to a personal computer.
At each site we used a triangular aquatic entomological net with a 30×30×30 cm mouth width and 0.5 mm mesh size to carry out a 2 m sweep at 1 m from shore and brushing the sediment with the bottom of the net, without collecting clay or rocks. Each sweep covered 0.6 m2 and all samples were taken by the same person. The macroinvertebrates found were collected and stored in 75% alcohol for later identification in the laboratory to the finest taxonomic level possible using the keys provided in Usinger (1956) and Triplehorn and Johnson (2005) for family level identifications, Berner and Hungerford (1977), and Ribeiro and Estévez (2009) for heteropterans, Friday (1988) for coleopterans, Harbach (1985) for Culicidae, Chapman (2007) for Amphipoda, Davies (1971) for Hirudinea, and Taylor (2003) for Gastropoda. They were treated as Recognizable Taxonomic Units (RTU’s) (Appendix). Although aquatic insects should be preserved in 95% or 100% alcohol, identifications were made within the following week of sampling, so they were preserved enough to avoid loss of taxonomically relevant structures.

We carried out 2 between-group principal components analyses (PCA), using as categories the different zones, and the different months, respectively, with the goal of identifying patterns in the ordering of sampling sites based on the variables evaluated. To prevent differences in the scale used for each variable from assigning higher relevance of some variables over others, the data were all scaled to a range of 0 to 1 using the following formula (Peshawa-Jamal & Rezhna-Hassan, 2014):
where x’ = value after scaling, x = observed value, min = minimum value recorded for that variable, and max = maximum value recorded for that variable. In the case of ORP, we used absolute values in the formula and a negative sign was assigned to the data to maintain the direction of the relationship.
To determine how macroinvertebrate communities are correlated with the concentration of the different parameters, we selected the RTU’s that contributed more than 5% of the total abundance to carry out a canonical correspondence analysis. The 5% criterion was arbitrary, but we considered that it allowed a cleaner picture by eliminating the species that had very few records. To do this, we used a matrix composed of each site in a different month as a new row. Thus, there was a total of 120 rows.
Finally, given that the residuals of most variables were not normally distributed and, instead, they were overdispersed, we constructed generalized linear model (Venables & Ripley, 2002). The dispersion of residuals was verified using the DHARMa package (Hartig, 2020), using R language version 3.6.1 (R Core Team, 2019). The best model was selected using the AIC criterion. The principal components and canonical correspondence analyses were done in Past software, version 3 (Hammer et al., 2001).
Because of a technical problem with the equipment, DO was not considered in the ordination analysis because we did not have these data for the month of July. However, it was considered in the generalized linear models. To do this, we did regressions contrasting only taxa vs. DO values, eliminating records of species from the month of July. The results, if significant, were included in the tables with the rest of the parameters.
Results
According to maximum permissible limits established by the Federal Law of National Water Rights (Conagua, 2019), the water of the lake does not meet all the standards required to be considered in 1 of the 4 water quality categories (source for public urban use, agricultural irrigation, protection for freshwater life, and protection for coastal and estuarine waters), on average over the course of the year (Table 1). However, the DO (during the months when it could be recorded) was above the minimum levels for any of the categories. The concentration of Zn was within the permissible levels of water quality for urban and irrigation use, while the concentration of Cu2+ was only acceptable for public urban use.
The first 2 PCA axes explained 94.6% of the system variation (Fig. 2). The most important correlations were positive: 68% with ORP, 44% with NH4+, 43% with P, and 41% with TDS. Although there was not a strict separation among the different sections of the lake, it was notable that zones I and II (both of which are toward the north) are mainly found on the left side of the map. This means that they have, in general, ORP values closer to zero and lower values of TDS, P, and NH4+. At the same time, the second component showed a 46% positive correlation with pH. However, in this component there were no apparent differences between zones. The rest of the parameters showed correlations below 30% with any of the components.
The PCA explained 90.7% of the system variation in the first 2 axes (Fig. 3). The most important positive correlation with the first axis was 46% with Cu2+, followed by a 45% correlation with pH. With respect to the negative correlations, the strongest was -53% with NO2-N. Therefore, the samples collected in July were characterized by higher NO2-N concentration and lower copper concentration and pH than the rest of the year.
The second component presented the strongest negative correlation, with -61% with Cu2+, and positive NH4+ and conductivity correlations of 50% and 30%, respectively. Although all months had points more or less distributed throughout the map, it is notable that October was the month with the highest ammonia and conductivity values.
Macroinvertebrate community. In total, we found 3,324 individuals belonging to 20 RTU’s, in at least 13 families (Appendix), which are found within 3 phyla (Arthropoda, Mollusca, and Annelida), and 4 classes (Insecta, Crustacea, Gastropoda, and Hirudinea). In the lake there were 4 orders of insects (Hemiptera, Diptera, Coleoptera, and Odonata), 1 crustacean order (Amphipoda), and 1 order of leech (Rynchobdellida). The most abundant species was Krizousacorixa femorata (Hemiptera: Corixidae), which contributed 1,424 individuals and was present in all 30 sites during at least 1 of the months, followed by Buenoa uhleri (Hemiptera: Notonectidae) with 1,085 individuals and presence in 29 sites. Three taxa had a single record during the sampling (Appendix).
The plot generated by the canonical correspondence analysis explained 89.02% of the variation in the data with the first 2 axes. In constructing it, we only considered K. femorata, B. uhleri, Chironomidae gen. sp. 1, Culiseta sp. 1, and Gastropoda gen. sp. 1 (Fig. 4).
The figure shows that K. femorata is found at higher abundance in sites with higher concentrations of DO, NO2-N, NO3-N, and Zn and elevated pH, ORP, and conductivity, but its abundance decreases with high concentrations of P, NH4+, TDS, and Cu2+. Buenoa uhleri had higher abundance in sites with relatively high concentrations of DO, NH4+ and P, as well as high values of ORP and pH, but its abundance decreased with higher values of NO2-N, NO3-N, conductivity, and Zn. Culiseta sp. 1, Gastropoda gen. sp. 2, and Chironomidae gen. sp. 1 were found in zones with relatively high concentrations of Cu2+, TDS, NH4+, and P, and low values of pH, ORP, DO, and Zn. In the generalized linear models, 4 of the most abundant species presented significant values, which are shown in Table 2.
Table 1
Characterization of the physico-chemical parameters of Lake Chalco (in mg/L, except for pH, conductivity, and ORP). Means and standard deviations are shown. Conditions that meet standards stipulated by the Federal Law of National Water Rights (“Ley Federal de Derechos en Materia de Aguas Nacionales”) for consideration within the named category are indicated with an asterisk (*). In all cases, except for dissolved oxygen, the number shows the maximum permissible level. The units are ppm, unless otherwise specified.
Physico-chemical parameters | Average | Source for public urban use | Agricultural irrigation | Protection for freshwater life | Protection for coastal and estuarine waters |
NH4+ | 5.45 ± 2.21 | – | – | 0.08 | 0.01 |
NO3-N | 4.28 ± 2.26 | 5 | – | – | 0.04 |
NO2-N | 7.38 ± 0.87 | 0.05 | – | – | 0.01 |
P | 3.32 ± 1.69 | 0.1 | 0.05 | 0.01 | |
Zn | 0.03 ± 0.01 | 5* | 2* | 0.02 | 0.02 |
Cu2+ | 0.54 ± 0.17 | 1* | 0.20 | 0.05 | 0.01 |
ORP (mV) | -253.25 ± 84.75 | – | – | – | – |
DO | 10.86 ± 2.01 | 4.0* | – | 5.0* | 5.0* |
TDS | 1,037.9 ± 473.3 | 500 | 500 | – | – |
Conductivity (µS/cm) | 2,126.35 ± 504.55 | – | – | – | – |
pH | 9.22 ± 0.27 | 6.0-9.0 | 6.0-9.0 | 6.5-8.5 | 6.0-9.0 |
Table 2
Coefficients from the GLM using a negative binomial error with species above 5% of abundance, for the significant parameters. Since the model is constructed using log as link function, the exponentiated value of the coefficient is shown to improve interpretability.
NH4+ | NO3-N | NO2-N | Cu2+ | ORP | DO | TDS | Conductivity | ||
K. femorata | Coefficient | -0.83 | – | – | – | – | 1.00 | -0.99 | – |
p | < 0.00 | – | – | – | – | < 0.00 | < 0.00 | – | |
B. uhleri | Coefficient | – | 1.05 | – | -0.15 | – | 1.01 | – | – |
p | – | 0.04 | – | 0.00 | – | 0.00 | – | – | |
Chironomidae gen. sp. 1 | Coefficient | -0.76 | -0.91 | 1.11 | – | – | – | – | 1.00 |
p | 0.00 | 0.03 | 0.04 | – | – | – | – | 0.00 | |
Culiseta sp. 1 | Coefficient | – | – | – | – | -0.99 | – | – | – |
p | – | – | – | – | 0.00 | – | – | – |
Discussion
Although there were differences between the most influential parameters among the 4 zones of the lake and months of the year, none of the zones shown presented acceptable quality characteristics according to the parameters established by the Federal Water Rights Law, so, no zones are recommended for anthropic use. Nevertheless, our results provide information of the zones and seasons of the year that requires attention in terms of management by the “ejidatarios”, in order to increase reasons to declare this area as a natural reserve. Our results are consistent with the data reported for other suburban water bodies in the Mexican Valley, such as lakes Texcoco, Xochimilco, and Zumpango, which are known to be turbid and meso- to eutrophic (Alcocer & Bernal-Brooks, 2010). The lacustrine zone of Lake Chalco has characteristics of an alkaline system with high conductivity, which, according to Caballero-Miranda (1997) has been present since at least 34,000 years ago. The elevated conductivity, at the same time, could correspond with a high TDS concentration.
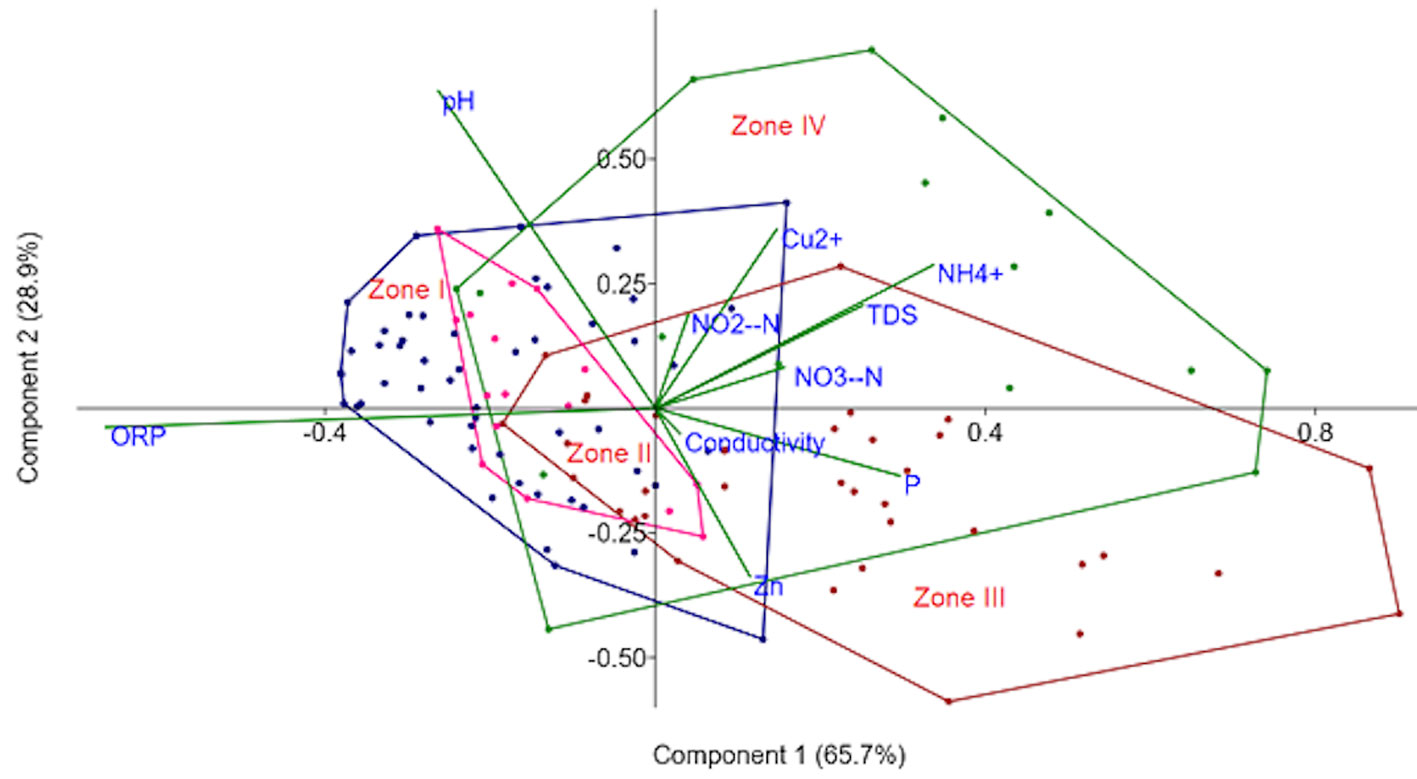
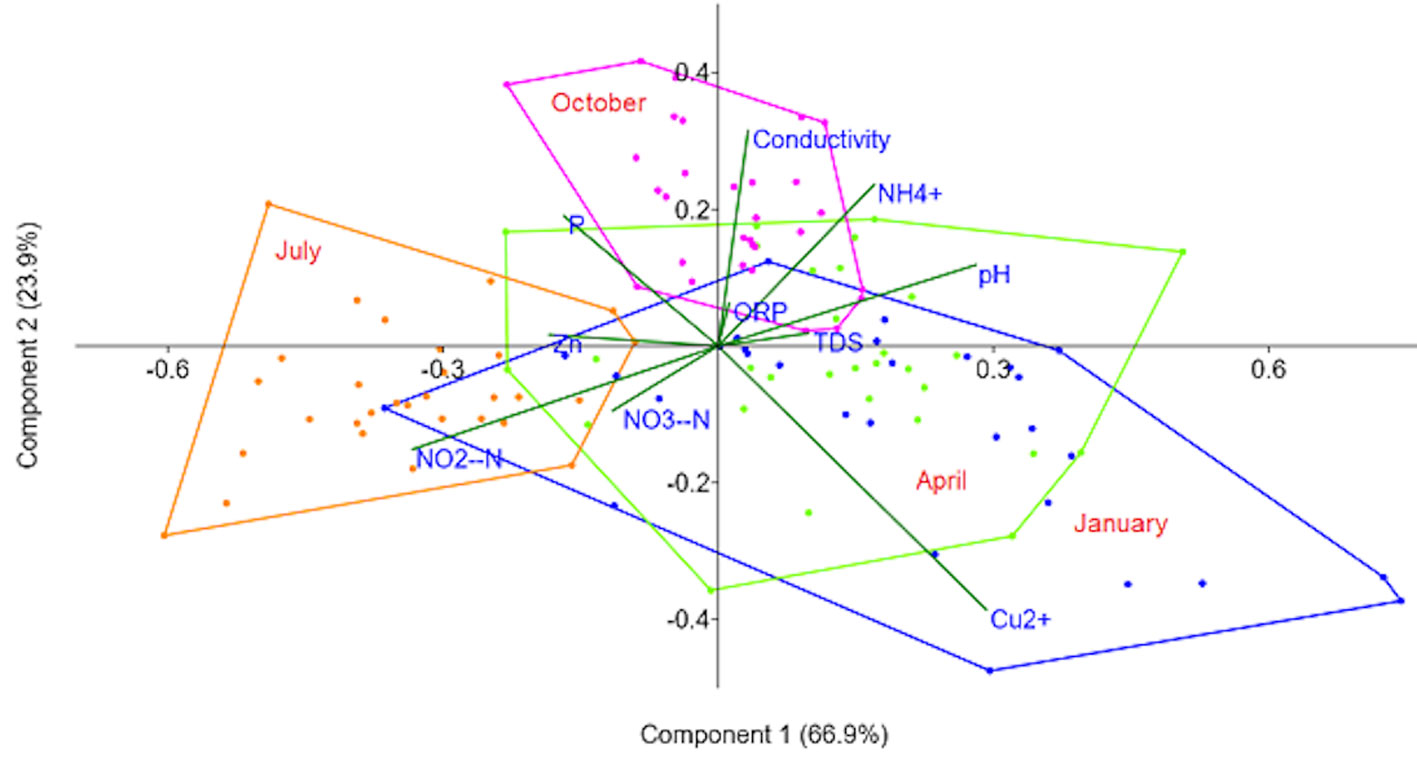
The southern portion of the lake (zones III and IV) had higher values of inorganic parameters, metals, conductivity, and TDS as well as larger negative values of ORP than the northern sections (zones I and II). This can be explained because the southern area receives more traffic from local people than the northern area, since it is bordered by both a highway and an area with pastures, crops, and landfills. There are even some small settlements whose wastewater is discharged into the lake.

For the consolidation of the seasonal map, only Cu2+, pH, NO2-N, NH4+, and conductivity had correlations above 30% with some of the axes. However, it is notable that some inorganic compounds, except NH4+, presented higher values in July than the other months. This could be explained because in Mexico City, farmers tend to sow crops in June (SIAP, 2018). Therefore, the use of different fertilizers in the area increases, some of which may include zinc. However, demonstrating that this is the cause of the increase in the metal is difficult, since the farmers were not aware of the composition of the supplements they used (Hernández-Juárez, 2019). If this were the case, the accumulation of nutrients in the water would be due to runoff after rain, given that this is the main source of the water in the lake (Ortiz & Ortega, 2007). At the same time, this would explain why June had the lowest quantity of the other parameters evaluated, since they would be dissolved in the larger volume of water. The fact that October is the month with the highest accumulation of NH4+ could be because in relative terms, it is the month with the largest negative ORP values, which facilitates the conversion of NO3-N to ammonia (Jørgensen, 1989). At the same time, it has higher electrical conductivity, which is consistent with the elevated TDS values. This would indicate that during October either particles flow into the lake or the particles present in the sediment are moved and suspended.
Two RTU’s accounted for 75.4% of abundance: K. femorata and B. uhleri, which may be explained by the absence of fishes that prey on these insects, at least in the sites where we collected. Since sampling sites did not include vegetation mats, there may be communities associated with T. latifolia that were not considered. Since there were no differences in water quality in the lake as a whole, no species can be considered as a strict bioindicator (Holt & Miller, 2010). Nevertheless, it was still possible to find significant relationships between some species and their associated physico-chemical parameters.
At least 16 RTU’s had some mechanism to avoid complete dependence on the oxygen dissolved in the water. Examples include the use of an air bubble by the hemipterans and coleopterans (Hungerford, 1919; Williams, 1936), the presence of hemoglobin in chironomids (Tichy, 1980), an air-breathing respiratory apparatus in culicids and sirfids (Dunavan, 1929), and lungs in mollusks of the genus Aplexa (Taylor, 2003). Exceptions included the leech, the dragonfly, and the amphipod. Although the prehispanic communities of the Mexican Basin used many resources (Alcocer & Bernal-Brooks, 2010), the records of the macroinvertebrates present at the time are imprecise. According to Alcocer & Escobar-Briones (1992), in the past, in that location there were at least 17 species of crustaceans, mollusks, and insects present, based on historical information.
The richness obtained in this study is poor (13 families) compared to other tropical lakes such as Montebello Lakes, with 40 (Cortés-Guzmán et al., 2019) to 47 families (Sosa-Aranda & Zambrano, 2020). Still, Chalco Lake’s richness is close to that of lakes located around central Mexico, where richness ranges from 4 (Metztitlan Lake; Juárez & Ibáñez, 2003) to 22 families (Lake Tecocomulco, Hidalgo State), where Corixidae represented more than 50% of the individuals (Rico-Sánchez et al., 2014). A case of special interest, due to their proximity, are those located in the remainders of prehispanic Xochimilco Lake, which are only about 8 km and had never been drained. A study of the macroinvertebrates associated to the invasive water hyacinth (Eichhornia crassipes) in Xochimilco Lake showed that there were 20 families of aquatic invertebrates (Rocha-Ramírez et al., 2014), some of which we also found in Chalco Lake. Although the way in which dispersion occurs in aquatic macroinvertebrates is not always clear (Bilton et al., 2001), we hypothesize that Chalco’s macroinvertebrate community is descended from that of Xochimilco given the more recent origin of the former.
The genus Krizousacorixa is endemic to Mexico and there are few studies of it (Hungerford, 1919). To date, most studies of corixids in the context of water quality in the country have been authored by Contreras-Rivero et al. (2001, 2005, 2008, 2012), and K. femorata has been present in all of them. However, the specific impact of physico-chemical parameters on the species has not been studied. As such, we consider that the present results give a more precise idea of the constraints on this species’ survival. It is not surprising that the evidence suggests that NH4+ is toxic to this species. However, it is interesting that this species’ abundance is negatively related to TDS, but not conductivity. This suggests that contrary to the data reported by Savage (1992), K. femorata is affected by the accumulation of particles more than conductivity. This could be because these particles restrict access to light, with negative consequences for this bug’s prey, ability to chase, or due to the decrease in sound propagation in turbid environments (Richards & Leighton, 2000) since this bug stridulates to communicate. In addition, this species is correlated with sites with high oxygen concentration even though all members of this family can use air bubbles to assist in respiration. However, it has been demonstrated that the volume of the bubble can be kept practically constant if the dissolved oxygen concentration of the water is higher than that of the air mixture, as long as nitrogen gas does not dissolve (Ege, 1915). As such, individuals that stay in a place with high oxygen concentration can spend hours underwater without needing to emerge to refill their bubble, unlike in less oxygenated areas (Thorpe, 1950).
The fact that B. uhleri responds positively to places with high NO3-N concentration is probably a response to a change in the conditions for the growth of its prey than to a physiological change, given the molecule’s low permeability of insect tissues (Jensen, 1996). It has been demonstrated, for example, that nitrate-rich environments can accelerate the reincorporation of organic matter into the environment (Meyer & Johnson, 1983), which could benefit basal levels of the trophic web. With respect to Cu2+, the L50 at 72 h has been calculated as 0.76 ppm for Notonecta glauca, another species from the same family, in 25 °C water (Dutta et al., 2011). However, in concentrations of this metal that allow survival, malformation in cells of the reproductive organs have been demonstrated in experiments with Anisops sardeus (Kheirallah, 2015). The explanation with respect to DO may be similar to what occurs in K. femorata, given that this family also uses bubbles to breathe air underwater.
With respect to the chironomids, it has been reported before that the total nitrogen concentration has particularly strong effects in some species (Odume & Muller, 2011). In this case, there was an inhibitory effect of NH4+ and NO3-N on the population of Chironomidae gen. sp. 1. At the same time, it shows that this family has wide variability in its physico-chemical affinities, since this RTU is correlated with high concentrations of NO2-N (while the lethal concentration for Chironomus piger and C. riparius is around 0.46 ppm (Neumann et al., 2001)), as well as high conductivity values. In terms of conductivity, specific intervals are correlated with different species of this family, such that different representatives have been recorded in lakes that range from 34 to 135,400 µS/cm (Eggermont et al., 2006). Finally, larvae of the genus Culiseta are correlated with ORP values near zero. This coincides with work by Chordá-Olmos (2014) that showed that the species of this genus present in Valencia, Spain, can be found in a range of -214 to 47 mV. However, they are most abundant in sites with values around -40 mV.
As usually occurs with water bodies located in urban areas, Lake Chalco shows a strong environmental stress that affects its biota. This could be a consequence of its historical management as well as its current use (Ortiz & Ortega, 2007). Moreover, its water quality is not good enough for anthropogenic use according to national laws.
Although a few species persist and appear to be resilient, there are no distinctions in water quality that permits the determination of bioindicators. Still, Lake Chalco emerges as an important refuge for local aquatic fauna.
Acknowledgements
To R. A. Farfán-Beltrán for elaboration of figure 1. To M. Hernández Quiroz and C. Ponce de León Hill for access to the equipment of the Environmental Analysis Unit.
Appendix. Species and morphospecies of macroinvertebrates collected in Lake Chalco.
Phyllum | Name | Classification | Jan | Apr | Jul | Oct |
Mollusca | Aplexa sp 1 | Hygrophyla: Physidae | 5 | 1 | 4 | 1 |
Gastropoda gen. sp. 1 | Not identified | 37 | 90 | 31 | 8 | |
Gastropoda gen. sp. 2 | Not identified | – | 8 | 37 | 1 | |
Annelida | Helobdella sp. 1 | Rynchobdellida: Glossiphoniidae | 1 | 7 | 6 | 12 |
Arthropoda | Crangonyctidae gen. sp. 1 | Amphipoda: Crangonyctidae | 1 | – | – | – |
Anax junius | Odonata: Aeshnidae | 1 | – | – | 1 | |
Belostoma aztecum | Hemiptera: Belostomatidae | 2 | – | 1 | – | |
Buenoa uhleri | Hemiptera: Notonectidae | 82 | 31 | 275 | 697 | |
Krizousacorixa azteca | Hemiptera: Corixidae | 173 | 117 | 709 | 425 | |
Hydroporus sp. 1 | Coleoptera: Dytiscidae | 1 | – | – | – | |
Helochares sp. 1 | Coleoptera: Hydrophylidae | 1 | – | – | – | |
Hydrophilidae sp. 1 | Coleoptera: Hydrophylidae | – | – | 1 | – | |
Rhantus sp. 1 | Coleoptera: Dytiscidae | 10 | – | – | 1 | |
Tropisternus sp. 1 | Coleoptera: Hydrophylidae | 2 | 0 | 1 | 1 | |
Culiseta sp. 1 | Diptera: Culicidae | 119 | 12 | 0 | 55 | |
Chironomidae gen. sp. 1 | Diptera: Chironomidae | 10 | 92 | 126 | 70 | |
Chironomidae gen. sp. 2 | Diptera: Chironomidae | 2 | 2 | 3 | 2 | |
Chironomidae gen. sp. 3 | Diptera: Chironomidae | 1 | 1 | 2 | 1 | |
Ephydridae gen. sp. 1 | Diptera: Ephrydidae | 10 | 0 | 5 | 2 | |
Syrphidae gen. sp. 1 | Diptera: Syrphidae | 4 | 2 | 3 | – |
References
Alcocer, J., & Bernal-Brooks, F. (2010). Limnology in Mexico. Hydrobiologia, 644, 15–68. https://doi.org/https://doi.org/
10.1007/s10750-010-0211-1
Alcocer, J., & Escobar-Briones, E. (1992). The aquatic biota of the now extinct lacustrine biota of the Mexico basin. Freshwater Forum, 2, 171–183.
Ayala-Pérez, V., Arce, N., & Carmona, R. (2013). Distribución espacio-temporal de aves acuáticas invernantes en la ciénega de Tláhuac, planicie lacustre de Chalco, México. Revista Mexicana de Biodiversidad, 84, 327–337. https://doi.org/
10.7550/rmb.28632
Bartram, J., Ballance, R., Organization, W. H., & Programme, U. N. E. (1996). Water quality monitoring: a practical guide to the design and implementation of freshwater quality studies and monitoring programs. In J. Bartram, & R. Balance (Eds.), E & F Spon. United Nations Environment Programme and the World Health Organization. https://apps.who.int/iris/handle/10665/41851
Berner, L., & Hungerford, H. B. (1977). The Corixidae of the Western Hemisphere. The Florida Entomologist, 60, 176. https://doi.org/10.2307/3493900
Bilton, D. T., Freeland, J. R., & Okamura, B. (2001). Dispersal in freshwater invertebrates. Annual Review of Ecology and Systematics, 32, 159–181. https://doi.org/https://doi.org/10.1146/annurev.ecolsys.32.081501.114016
Caballero-Miranda, M. (1997). Reconstrucción paleolimnológica del lago de Chalco, México, durante el último máximo glaciar: el registro de las diatomeas entre 34,000 y 15,000 años A.P. Revista Mexicana de Ciencias Geológicas, 14, 91–100.
Chapman, J. (2007). Gammaridea. In J. Carlton (Ed.), The Light and Smith manual (4th Ed.) (pp. 545–618). Richmond: University of California Press.
Chordá-Olmos, F. A. (2014). Biología de mosquitos (Diptera: Culicidae) en enclaves representativos de la Comunidad Valenciana. Valencia: Universitat de Valencia.
Contreras-Rivero, G., Camarillo-de la Rosa, G., Navarrete-Salgado, N. A., & Elías-Férnandez, G. (2005). Corixidae (Hemiptera Heteroptera) en el lago urbano del parque Tezozomoc, Azcapotzalco, México, D.F. Revista Chapingo Serie Ciencias Forestales y del Ambiente, 11, 93–97.
Contreras-Rivero, G., Figueroa-Abundiz, V. A., Martínez-Téllez, A., Navarrete-Salgado, N. A., Hiotrón-Labrada, E., Arteaga-Garrido, P. M. et al. (2012). Primeros registros de la familia Corixidae (Hemiptera) en el embalse Macua, Estado de México. Investigación y Ciencia, 20, 61–63.
Contreras-Rivero, G., Navarrete-Salgado, N. A., Elías-Férnandez, G., & Rojas Bustamante, M. L. (2001). Aspectos ecológicos de los Corixidae (Hemiptera, Heteroptera) en el estanque piscícola “GL” de Soyaniquilpan de Juárez, Estado de México. Hidrobiológica, 11, 153–160.
Contreras-Rivero, G., Navarrete-Salgado, N. A., & Lara-Vázquez, J. Á. (2008). Aquatic hemiptera into two piscicole ponds in Estado de Mexico. Revista Chapingo Serie Horticultura, 14, 39–43.
Cortés-Guzmán, D., Alcocer, J., & Oseguera, L. A. (2019). Benthic macroinvertebrate community diversity of Montebello
Lakes, Chiapas. Revista Mexicana de Biodiversidad, 90, e902769. https://doi.org/10.22201/ib.20078706e.2019.90.2769
Davies, R. W. (1971). A key to the freshwater Hirudinoidea of Canada. Journal of the Fisheries Research Board of Canada, 28, 543–552. https://doi.org/10.1139/f71-077
Department of Environmental Protection (Maine). (2014). Protocols for sampling aquatic macroinvertebrates in freshwater wetlands. Portland, ME: Government of the State of Maine.
Domínguez-Rubio, I. (1997). Caracterización de los suelos. In M. T. Barreiro-Güemes, R. Sánchez-Trejo, A. Aguirre-León, & L. A. Ayala-Pérez (Eds.), Ecología del humedal de San Pedro Tláhuac: un sistema lacustre del Valle de México (pp. 17–30). Ciudad de México: Universidad Autónoma Metropolitana.
Dunavan, D. (1929). A study of respiration and respiratory organs of the Rat-tailed Maggot, Eristalis arbustorum L. (Diptera: Syrphidæ). Annals of the Entomological Society of America, 22, 731–753. https://doi.org/10.1093/aesa/22.4.731
Dutta, A., Dutta, S., & Kumari, S. (2011). Temperature – dependent toxicokinetics of copper and lead in Notonecta glauca L. (Hemiptera: Notonectidae) under laboratory condition. Journal of Advanced Laboratory Research in Biology, 2, 35–39.
Ege, R. (1915). On the respiration function of the air stores carried by some aquatic insects (Corixidae, Dytiscidae and Notonecta). Zeitschrift Für Allgemeine Physiologie, 17, 81–124.
Eggermont, H., Heiri, O., & Verschuren, D. (2006). Fossil Chironomidae (Insecta: Diptera) as quantitative indicators of past salinity in African lakes. Quaternary Science Reviews, 25, 1966–1994. https://doi.org/10.1016/j.quascirev.2005.04.011
Friday, L. E. (1988). A key to the adults of British water beetles, Vol. 189. Preston Montford: Field Studies Council Publications.
Hammer, Ø., Harper, D., & Ryan, P. (2001). Past: Paleontological Statistics Software Package for Education and Data Analysis. Palaeontologica Electronica, 4, 9.
Harbach, R. E. (1985). Pictorial keys to the genera of mosquitoes, subgenera of Culex and the species of Culex (Culex) occurring in southwestern Asia and Egypt, with a note on the subgeneric placement of Culex deserticola (Diptera, Culicidae). Mosquito Systematics, 17, 83–107.
Hartig, F. (2020). DHARMa: Residual Diagnostics for Hierarchical (Multi-Level/Mixed) Regression Models (0.3.0).
Hernández-Juárez, N. L. (2019). Análisis de las condiciones socio-agrícolas en la zona circunvecina al nuevo lago de Chalco. Ciudad de México: Universidad Nacional Autónoma de México.
Holt, E., & Miller, S. (2010). Bioindicators: using organism to measure environment impacts. Nature Education Knowledge, 3, 8.
Hungerford, H. B. (1919). The biology and ecology of aquatic and semi-aquatic Hemiptera. Cornell: Cornell University.
Jeanmougin, M., Leprieur, F., Loïs, G., & Clergeau, P. (2014). Fine-scale urbanization affects Odonata species diversity in ponds of a megacity (Paris, France). Acta Oecologica, 59, 26–34. https://doi.org/10.1016/j.actao.2014.05.008
Jensen, F. B. (1996). Uptake, elimination and effects of nitrite and nitrate in freshwater crayfish (Astacus astacus).
Aquatic Toxicology, 34, 95–104. https://doi.org/10.1016/0166-445X(95)00030-8
Johnson, D. L., Ambrose, S. H., Bassett, T. J., Bowen, M. L., Crummey, D. E., Isaacson, J. S. et al. (1997). Meanings of
environmental terms. Journal of Environment Quality, 26, 581–589. https://doi.org/10.2134/jeq1997.0047242500
2600030002x
Jørgensen, K. S. (1989). Annual pattern of denitrification and nitrate ammonification in estuarine sediment. Applied and Environmental Microbiology, 55, 1841–1847. https://doi.org/10.1128/aem.55.7.1841-1847.1989
Juárez, J., & Ibáñez, A. (2003). Abundancia y primer registro de macroinvertebrados bentónicos en el lago de Metztitlán, Hidalgo, México. Hidrobiológica, 13, 137–144.
Kheirallah, D. A. (2015). Ultrastructure biomarker in Anisops sardeus (Heteroptera: Notonectidae) for the assessment and monitoring of water quality of Al-Mahmoudia Canal, western part of Nile Delta, Egypt. Journal of Bioscience and Applied Research, 1, 2356–9182.
Knop, E. (2016). Biotic homogenization of three insect groups due to urbanization. Global Change Biology, 22, 228–236. https://doi.org/10.1111/gcb.13091
Kozlowski, G., & Bondallaz, L. (2013). Urban aquatic ecosystems: Habitat loss and depletion of native macrophyte diversity during the 20th century in four Swiss cities. Urban Ecosystems, 16, 543–551. https://doi.org/10.1007/s11252-012-0284-x
McDonald, R. I., Marcotullio, P. J., & Güneralp, B. (2013). Urbanization and global trends in biodiversity and ecosystem services. In T. Elmqvist, M. Fragkias, J. Goodness, B. Güneralp, P. J. Marcotullio, R. I. McDonald et al. (Eds.), Urbanization, biodiversity and ecosystem services: challenges and opportunities (pp. 31–52). Netherlands: Springer. https://doi.org/10.1007/978-94-007-7088-1_3
Meyer, J. L., & Johnson, C. (1983). The influence of elevated nitrate concentration on rate of leaf decomposition in a stream. Freshwater Biology, 13, 177–183. https://doi.org/10.1111/j.1365-2427.1983.tb00669.x
Monteiro-Junior, C. D. S., Juen, L., & Hamada, N. (2014a). Analysis of urban impacts on aquatic habitats in the central Amazon basin: Adult odonates as bioindicators of environmental quality. Ecological Indicators, 48, 303–311. https://doi.org/10.1016/j.ecolind.2014.08.021
Monteiro-Júnior, C. S., Juen, L., & Hamada, N. (2014b). Effects of urbanization on stream habitats and associated adult dragonfly and damselfly communities in central Brazilian Amazonia. Landscape and Urban Planning, 127, 28–40. https://doi.org/10.1016/j.landurbplan.2014.03.006
Neumann, D., Kramer, M., Raschke, I., & Gräfe, B. (2001). Detrimental effects of nitrite on the development of benthic Chironomus larvae, in relation to their settlement in muddy sediments. Archiv Für Hydrobiologie, 153, 103–128. https://doi.org/10.1127/ARCHIV-HYDROBIOL/153/2001/103
Norris, R. H., & Morris, K. R. (1995). The need for biological assessment of water quality: Australian perspective. Austral Ecology, 20, 1–6. https://doi.org/10.1111/j.1442-9993.1995.tb00516.x
Odume, O. N., & Muller, W. J. (2011). Diversity and structure of Chironomidae communities in relation to water quality differences in the Swartkops River. Physics and Chemistry of the Earth, 36, 929–938. https://doi.org/10.1016/j.pce.2011.07.063
Oertli, B. (2008). The use of dragonflies in the assessment and monitoring of aquatic habitats. In A. Córdoba-Aguilar (Ed.), Dragonflies and damselflies: model organisms for ecological and evolutionary research (pp. 79–96). Oxford: Oxford University Press. https://doi.org/10.1093/acprof:oso/9780199230693.003.0007
Oleson, D. (2013). Macroinvertebrates as indicators of water quality. Philadelphia: University of Pennsylvania. http://extension.psu.edu/natural-resources/water/news/2013/macroinvertebrates-as-indicators-of-water-quality
Ortiz, D., & Ortega, A. (2007). Origin and evolution of a new lake in the Chalco plain: implications for land subsidence and flooding hazards to the urban areas of Valle de Chalco (State of Mexico) and Tláhuac (Federal District). Boletín del Instituto de Geografía, UNAM, 64, 26–42.
Paoletti, M. G. (1999). Using bioindicators based on biodiversity to assess landscape sustainability. Agriculture, Ecosystems and Environment, 74, 1–18. https://doi.org/10.1016/S0167-8809(99)00027-4
Peshawa-Jamal, M. A., & Rezhna-Hassan, F. (2014). Data normalization and standardization. A technical report. Machine Learning Technical Reports, 1, 1–6.
R Core Team. (2019). R: A language and environment for statistical computing. R Foundation for Statistical Computing, Vienna, Austria. https://www.R-project.org/
Resh, V. H., & Unzicker, J. D. (1975). Water quality monitoring and aquatic organisms: the importance of species identification. Water Pollution Control Federation, 47, 9–19.
Ribeiro, J. R. I., & Estévez, A. L. (2009). The small species of Belostoma Latreille (Heteroptera, Belostomatidae). III. A revision of oxyurum group, with a new species from Brazil and description of the male of B. noualhieri Montandon. Revista Brasileira de Entomologia, 53, 207–215. https://doi.org/10.1590/S0085-56262009000200004
Richards, S. D., & Leighton, T. G. (2000). Sonar performance in turbid and bubbly environments. The Journal of the Acoustical Society of America, 108, 2562–2562. https://doi.org/10.1121/1.4743513
Rico-Sánchez, A. E., Rodríguez-Romero, A. J., López-López, E., & Sedeño-Díaz, J. E. (2014). Patrones de variación espacial y temporal de los macroinvertebrados acuáticos en la Laguna de Tecocomulco, Hidalgo (México). Revista de Biología Tropical, 62, 81–96. https://doi.org/10.15517/rbt.v62i0.15780
Robles-Palacios, K. M. (2018). Modelo de interconexión entre la calidad del agua y la atmósfera en el Nuevo Lago de Chalco, México (Bachelor Thesis). Ciudad de México: Universidad Nacional Autónoma de México.
Rocha-Ramírez, A., Robles-Valderrama, E., & Ramírez-Flores, E. (2014). Invasive alien species water hyacinth Eichhornia crassipes as abode for macroinvertebrates in hypertrophic Ramsar Site, Lake Xochimilco, Mexico. Journal of Environmental Biology, 35, 1071–1080.
Samways, M. J., & Steytler, N. S. (1996). Dragonfly (Odonata) distribution patterns in urban and forest landscapes, and recommendations for riparian management. Biological Conservation, 78, 279–288. https://doi.org/10.1016/S00063207(96)00032-8
Savage, A. A. (1992). The distribution of Corixidae in relation to the water quality of British Lakes: a monitoring model. Freshwater Biodiversity and Water Quality, 4, 32–61.
Sedesol (Secretaría de Desarrollo Social). (2011). Atlas de riesgo municipio de Valle de Chalco, Solidaridad. Informe final. Ayuntamiento de Valle de Chalco. Consultado en línea en: http://rmgir.proyectomesoamerica.org/PDFMunicipales/2011/vr_15122_AR_Valle_Chalco.pdf
Servín, C. C. (2005). Las sequías en México durante el siglo XIX. Investigaciones Geográficas, 56, 118–133.
SIAP (Servicio de Información Agroalimentaria y Pesquera). (2018). Fecha de siembras y cosechas en México.
https://www.gob.mx/siap/articulos/fecha-de-siembras-y-cosechas-en-mexico?idiom=es
Sosa-Aranda, I., & Zambrano, L. (2020). Relationship between turbidity and the benthic community in the preserved Montebello Lakes in Chiapas, Mexico. Marine and Freshwater Research, 71, 824–831. https://doi.org/10.1071/MF19090
Taylor, D. W. (2003). Introduction to Physidae (Gastropoda: Hygrophila); biogeography, classification, morphology. Revista de Biologia Tropical, 51 Suppl 1, 1–263, 265–287.
Thorpe, W. H. (1950). Plastron respiration in aquatic insects. Biological Reviews, 25, 344–390. https://doi.org/10.1111/j.1469-185X.1950.tb01590.x
Tichy, H. (1980). The haemoglobin synthesising tissue of Chironomus. In D. A. Murray (Ed.), Chironomidae, ecology, systematics, cytology, and physiology (pp. 43–50). Pergamon: Elsevier. https://doi.org/10.1016/B978-0-08-025
889-8.50011-8
Triplehorn, C., & Johnson, N. (2005). Borror and DeLong’s Introduction to the study of insects, 7th edition. Belmont, California: Thomson Learnings.
Usinger, R. L. (1956). Aquatic insects of California, with keys to North American genera and California species. Berkeley: University of California Press. https://doi.org/10.5962/bhl.title.61952
Venables, W. N., & Ripley, B. D. (2002). Modern applied statistics with S. New York: Springer.
Villalobos-Jiménez, G., Dunn, A. M., & Hassall, C. (2016). Dragonflies and damselflies (Odonata) in urban ecosystems: A review. European Journal of Entomology, 113, 217–232. https://doi.org/10.14411/eje.2016.027
Williams, F. X. (1936). Biological studies in Hawaiian water-loving insects, Part 1: Coleoptera or Beetles, Part 2: Odonata or Dragonflies. Proceedings of the Hawaiian Entomological Society, 9, 235–349.