Ivonne Trejo-Ventura, Gema Armendáriz, José Luis Villalobos, Fernando Álvarez *
Universidad Nacional Autónoma de México, Instituto de Biología, Colección Nacional de Crustáceos, Tercer circuito s/n, Ciudad Universitaria, 04510 Ciudad de México, Mexico
*Corresponding author: falvarez@ib.unam.mx (F. Álvarez)
Received: 24 February 2023; accepted: 13 June 2023
Abstract
The setal arrangement on the dactyl and propodus of pereopods 1 and 2, defined here as the feeding apparatus, of 3 species of cave shrimps of the genus Typhlatya (T. mitchelli, T. pearsei, T. dzilamensis) from the Yucatán Peninsula was studied using SEM micrographs. The setae were classified according to their morphology and position in the propodus and dactyl resulting in 6 main types. The 3 Typhlatya species differed slightly in the number of each type of setae and showed some morphological variations, especially in the central setae. A comparison of the setal arrangement of Typhlatya spp. with that of the epigean Potimirim mexicana and P. glabra, showed important differences probably driven by the specialization to specific habitats.
Keywords: Typhlatya mitchelli; Typhlatya pearsei; Typhlatya dzilamensis; Potimirim mexicana; Potimirim glabra
© 2023 Universidad Nacional Autónoma de México, Instituto de Biología. This is an open access article under the CC BY-NC-ND license
(http://creativecommons.org/licenses/by-nc-nd/4.0/).
Morfología del aparato de alimentación de los camarones de cueva del género Typhlatya (Decapoda: Atyidae) de la península de Yucatán y comparación con especies epigeas
Resumen
A través de micrografías obtenidas del MEB se estudió el arreglo de las cerdas en el dactilo y propodio de los pereiópodos 1 y 2, definido aquí como el aparato de alimentación, de 3 especies de camarones de cueva del género Typhlatya (T. mitchelli, T. pearsei, T. dzilamensis) de la península de Yucatán. Las setas se clasificaron de acuerdo con su morfología y posición en el propodio y dactilo resultando 6 tipos principales. Las 3 especies de Typhlatya difirieron ligeramente en el número de cada tipo de seta y mostraron algunas variaciones morfológicas, especialmente en las setas centrales. Una comparación del arreglo setal de Typhlatya spp. con el de las especies epigeas Potimirim mexicana y P. glabra, mostró diferencias importantes generadas probablemente por la especialización a un determinado hábitat.
Palabras clave: Typhlatya mitchelli; Typhlatya pearsei; Typhlatya dzilamensis; Potimirim mexicana; Potimirim glabra
© 2023 Universidad Nacional Autónoma de México, Instituto de Biología. Este es un artículo Open Access bajo la licencia CC BY-NC-ND
Introduction
The shrimps of the family Atyidae have been the subject of several functional morphology studies due to the singular chelar structure of their first 2 pairs of pereopods (Felgenhauer & Abele, 1983, 1985; Fryer, 1977) (Fig. 1). The characteristic cheliped fans compose a feeding apparatus that is oriented against the flow in lotic environments to capture food particles and can also be used for scraping the substrate for attached small epiphytic organisms in pools or bodies of water with no current (Bailey-Brock & Brock, 1993; Felgenhauer & Abele, 1983). In epigean atyid species other morphological and behavioral adaptations complement this feeding mode, such as the orientation of the organism against the current and the strong pereopods 3-5 with claw-like dactyli that help the organism hold its position.
Within the Atyidae, the genus Typhlatya comprises 18 cave dwelling species distributed in coastal areas in the Greater Caribbean, eastern Pacific and mid-Atlantic islands, the Mediterranean and in Zanzibar, off the east coast of Africa (Alvarez et al., 2005; Jurado-Rivera et al., 2017). Shrimps of the genus Typhlatya have specialized to scrape rather than to filter out particles due to the oligotrophic, nutrient poor water inside anchialine caves, and to the usually low flows inside them (Beddows et al., 2007). The way in which Typhlatya shrimps feed has recently become of great interest due to the finding that they may be feeding on bacteria, both heterotrophic and chemoautotrophic, as well as on other food items (Brankovits et al., 2017; Chávez-Solís et al., 2020), thus redefining how the anchialine food web may be structured. Further, all species of Typhlatya are stygobitic and may also be using the chelipedal setae to sense the substrate and type of food where they dwell. Despite the growing interest in these shrimps there are no in-detail descriptions of the setal arrangement in the chelae of Typhlatya.
With the goal of determining how the feeding apparatus is structured in Typhlatya shrimps, here we describe, based on SEM micrographs, the setal types on the chelipeds of 3 species from the Yucatán Peninsula. We adopt the scheme proposed by Garm and Watling (2013) who classified the crustacean setae into 7 main types according to their morphology and function: pappose, plumose, composite, serrate, papposerrate, simple and cuspidate. But as will be treated later, we identified some variations of the basic types already described. To make comparisons among species we distinguish different areas of the internal surface of both dactyl and propodus of the first 2 pereopods (P1, P2), as shown in figure 2. Further, to establish a reference point we examined the setal types and their arrangement on the chelipeds of the epigean Potimirim glabra and P. mexicana.
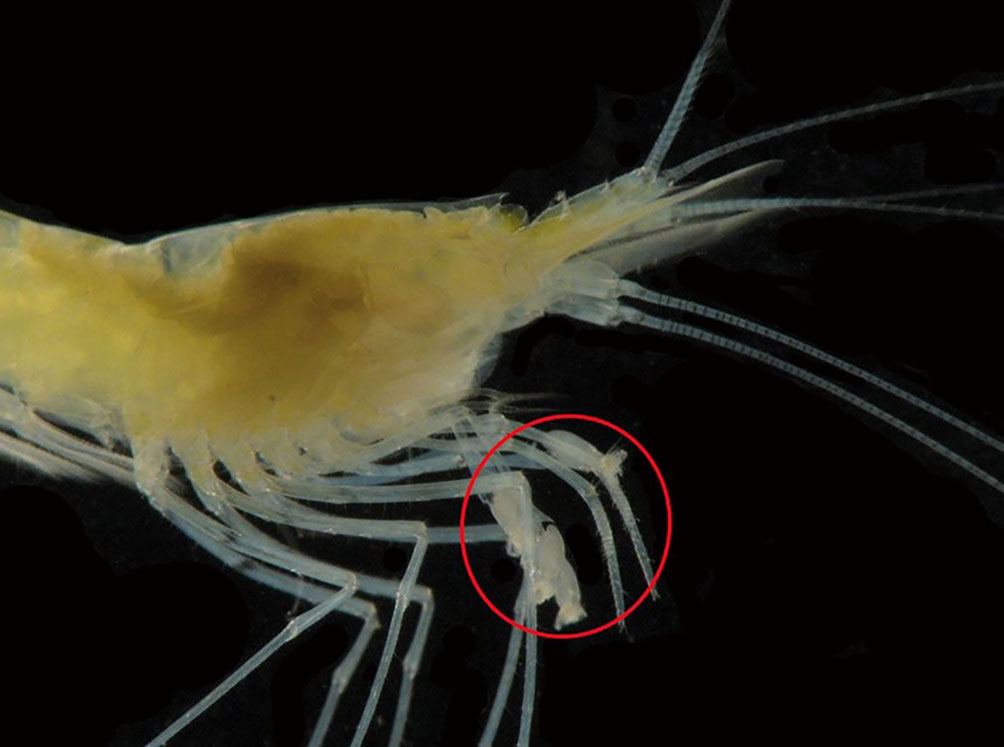
Materials and methods
All specimens were obtained from samples deposited in the Colección Nacional de Crustáceos (CNCR) of the Instituto de Biología, Universidad Nacional Autónoma de México (UNAM). Species and collection data are as follows. Typhlatya mitchelli: Cenote Chooj Ha (20.635º N, 89.612º W), Yucatán, Mexico, 26/06/2015, CNCR 33055; Cenote Vaca Ha (20.210° N, 87.501° W), Quintana Roo, Mexico, 3/02/2019, CNCR 32906. Typhlatya pearsei: Cenote Huhunpiit (21.187° N, 88.648° W), Yucatán, Mexico, 9/10/2016, CNCR 33481; Cenote Vaca-Ha (20.210° N, 87.501° W), Quintana Roo, Mexico, 25/06/2015, CNCR 33036. Typhlatya dzilamensis: Cenote Na´ach Wennen Ha (20.168° N, 87.456° W), Quintana Roo, Mexico, 3/08/2013, CNCR 28389. Potimirim glabra, Río Murga (17.694° N, 100.672° W), Guerrero, Mexico, 16/07/1984, CNCR 2686. Potimirim mexicana, Cosamaloapan (18.394° N, 94.189° W), Veracruz, Mexico, 28/03/1956, CNCR 1897. For the former 2 species, specimens from Yucatán and Quintana Roo that are ca. 350 km apart are presented. All organisms were fixed in 70% EtOH, in the field.
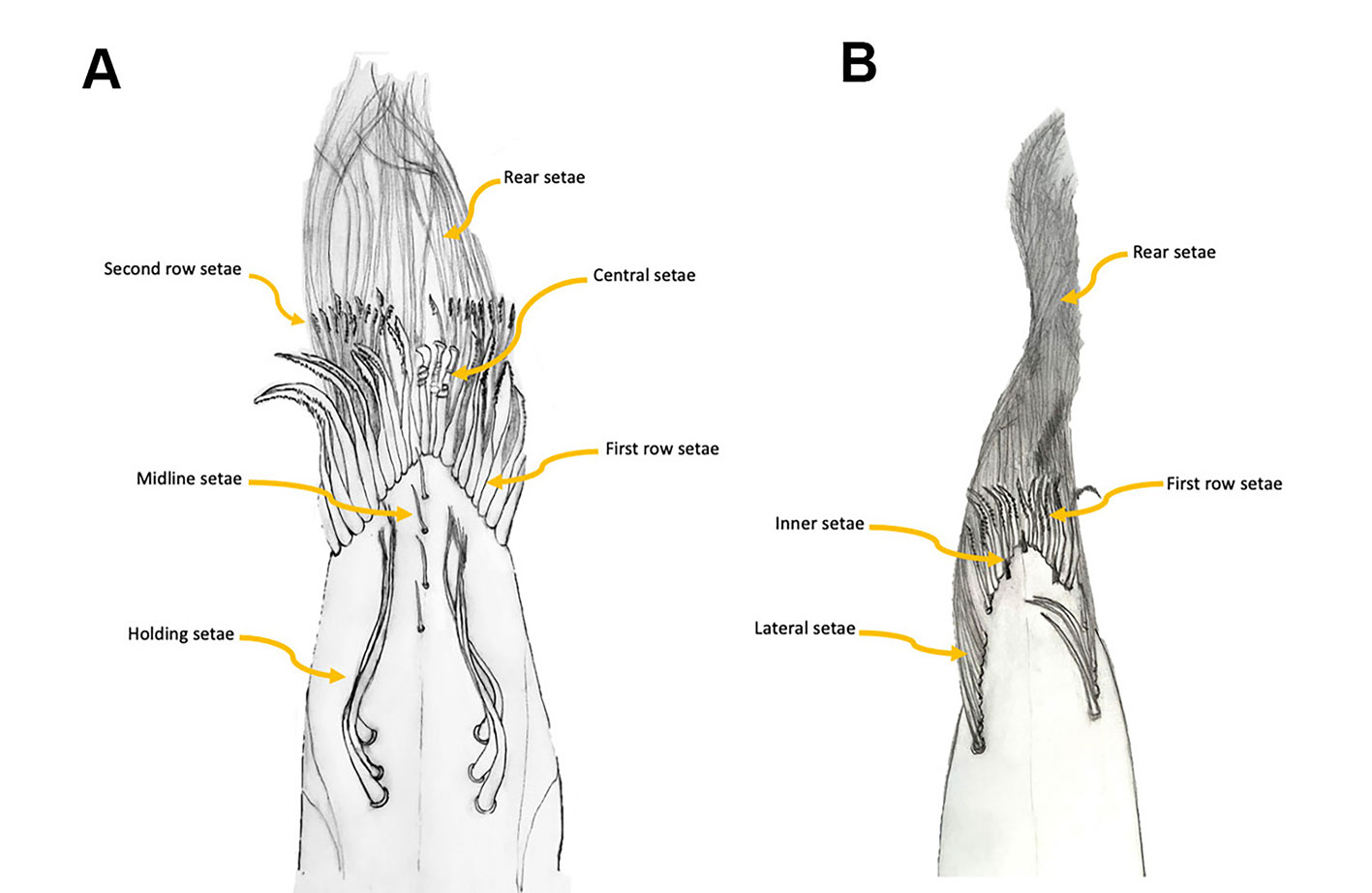
After being identified to species level, each specimen was dissected under a dissecting microscope to remove the first 2 pairs of pereopods. The pereopods were then rehydrated through successive changes of 15 min each in EtOH at 100%, 96%, 70%, 50%, 30%, 10%, and distilled water. The pereopods were then cleaned by submerging them for 4 min (with one repetition) in an ultrasound bath in a Branson 2000 sonicator with a light soap solution. After rinsing with distilled water, the samples were progressively dehydrated to 70% EtOH. At this point they were placed in a 1:1 anhydrous glycerol – 70% EtOH solution for 1 day to complete the removal of any remaining debris in the setae. The pereopods were rinsed 3 times with 70% EtOH before completing the dehydration in 100% EtOH.
The samples were dried placing them for 10 min in 3 different solutions of 100% EtOH/acetone: 3/1, 3/3 and 1/3. The chelae were dissected separating the dactyl from the propodus and attaching them separately to SEM stubs. The samples were then gold coated in a Quorum Q150 R ES coater. We used a Hitachi SU1510 scanning electron microscope at the Laboratorio de Microscopía de la Biodiversidad at Instituto de Biología, UNAM, to observe and photograph the samples. Photographs were taken of the internal surface of the dactyl and propodus of the first and second pereopods of Typhlatya mitchelli, T. pearsei, T. dzilamensis, Potimirim glabra, and P. mexicana.
We describe the types of setae present in each propodus and dactyl based on 2 individuals of each species. The lateral holding setae, the setae along the midline of the internal surface, the first and second row setae, and the rear setae were measured and counted (Fig. 2). The ecological implications of the observed setal types are discussed and a comparison with related epigean species is presented.
Results
The first finding is that the setal arrangement in all 3 Typhlatya species is similar in dactyl and propodus and in pereopod 1 (P1) and pereopod 2 (P2), so the following descriptions apply to all 3 species, with species-specific details mentioned for each one. The feeding apparatus in the chelae comprises different kinds of setae that serve different functions: filtering, scraping, sensing. All the setae described have an infracuticular articulation, or deep sockets (Garm, 2004), suggesting that they all are innervated. The 3 Typhlatya species show in P1 and P2, from the inner midline of the propodus and dactyl, and from the proximal to the distal end, 6 basic types of setae according to their function (Fig. 2A): holding setae, presumably to hold the dactyl and propodus as the chela opens and closes (Figs. 3, 4F); midline setae, with a probable sensory function (Figs. 3, 4C-E, 5); first-row setae, used for scraping (Figs. 3, 4G, H); central setae, used for scraping and probably holding to the substrate (Figs. 3, 5A-C); second-row setae, used for scraping and capturing small particles (Figs. 3, 5D, E); and rear setae, which filter out small particles (Figs. 3, 5F).
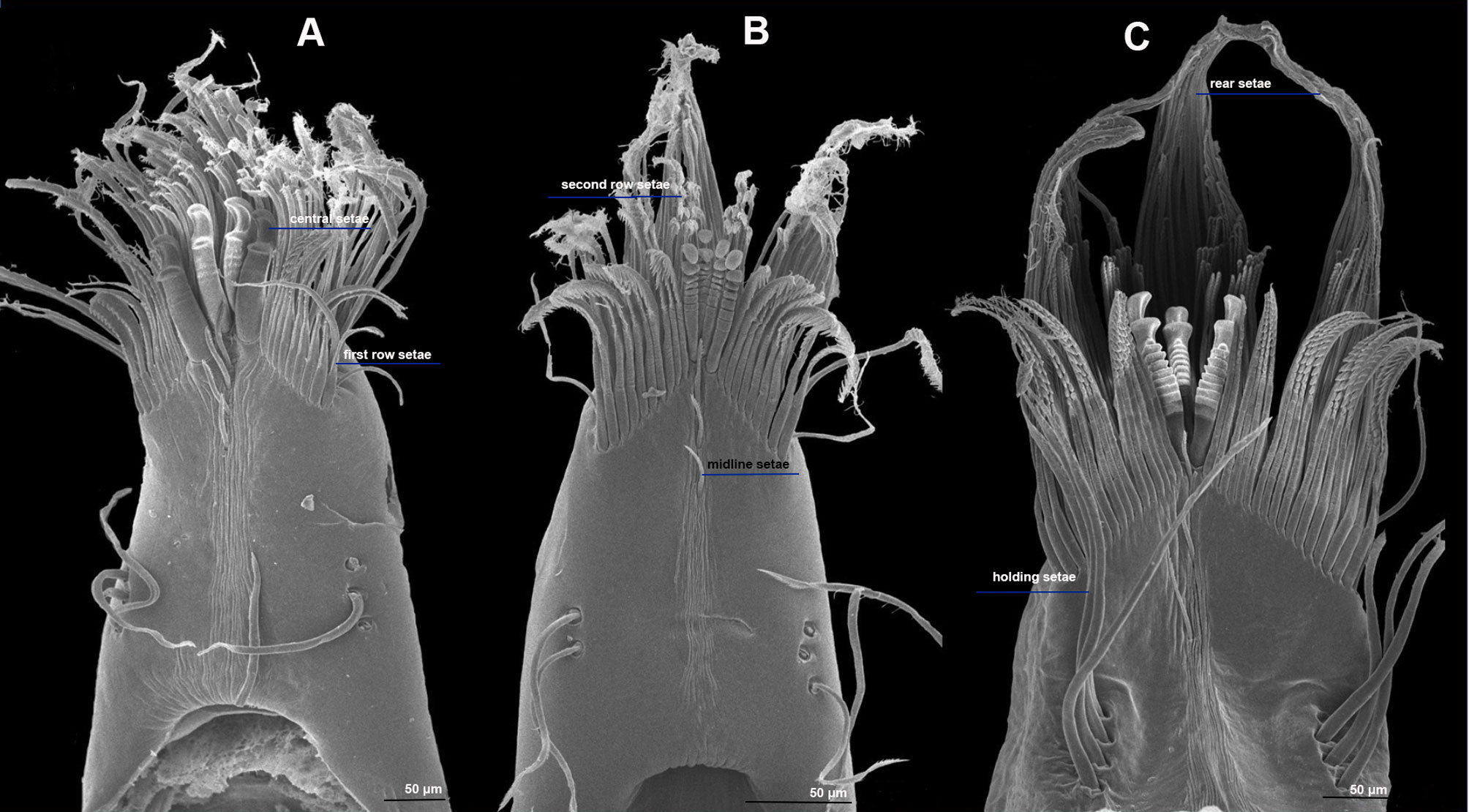
The holding setae are situated apart from the apical group of setae; according to Garm (2004), these would be pappose setae. They are simple for most of their length developing scattered simple setules apically (Fig. 4F), their length varies from 150 mm in T. pearsei to 250 mm in T. dzilamensis (Fig. 3A-C). Their number may vary from 2 on the left side and 2 on the right side of the article, to 4 and 4 setae, respectively. The observed variation in the number of setae in the dactyl and propodus of P1 and P2 of the 3 Typhlatya species is shown in figure 7. Typhlatya mitchelli has the fewer setae of this type (x = 2.75 per side, n = 8), T. pearsei is intermediate (x = 3.12 per side, n = 8) and T. dzilamensis has more (x = 3.5 per side, n = 4).
The number midline setae, in the distal portion of the dactyl and propodus, vary from 3 to 5; they are short (~ 45 mm long), slender, with 2 rows of minute setules on the distal portion and a bifid apex (Fig. 4C-E). At a closer inspection, in some of these setae a small subapical pore can be seen (Fig. 6). These can be classified as serrulate setae (Garm, 2004).
The first-row setae have a serrate inner surface along the distal half, with the strong teeth are directed proximally (Figs. 3, 4G, H). In T. pearsei and T. dzilamensis the proximal half of the inner surface of these setae are covered with minute excrescences (Fig. 4G, H). These setae increase in size from the central to the lateral portion; in T. mitchelli their size range from 94.5 to 104.2 mm (n = 84 setae), in T, pearsei from 61.3 to 91.2 mm (n = 81 setae), and in T. dzilamensis from 125.8 to 145.4 mm (n = 66 setae). Typhlatya mitchelli and T. pearsei showed from 19 to 22 setae (n = 4 specimens/species), and T. dzilamensis 32 to 34 (n = 2 specimens) (Fig. 7).
The central setae (Figs. 3, 5A-C) are stout, thickly lamellate, could be used to scrape or to hold the cheliped in position; they have a strong hook-like appearance apically. These setae don´t seem to have been described before, and therefore, we will call them lamellate setae. They vary from being very thick in T. mitchelli to very slender in some T. pearsei, and also vary in the number and thickness of the lamellae, from none in T. mitchelli to 7 in T. dzilamensis (Fig. 5A-C). In all the 3 species studied there are 3 or 4 central setae; however, they differ in size: T. mitchelli, x = 104.3 mm (n = 4); T. pearsei, x = 54.6 mm (n = 4); and T. dzilamensis, x = 126.4 mm (n = 3). Typhlatya pearsei is the one species that shows the greatest variation in these setae (Fig. 3B vs. Fig. 5B).
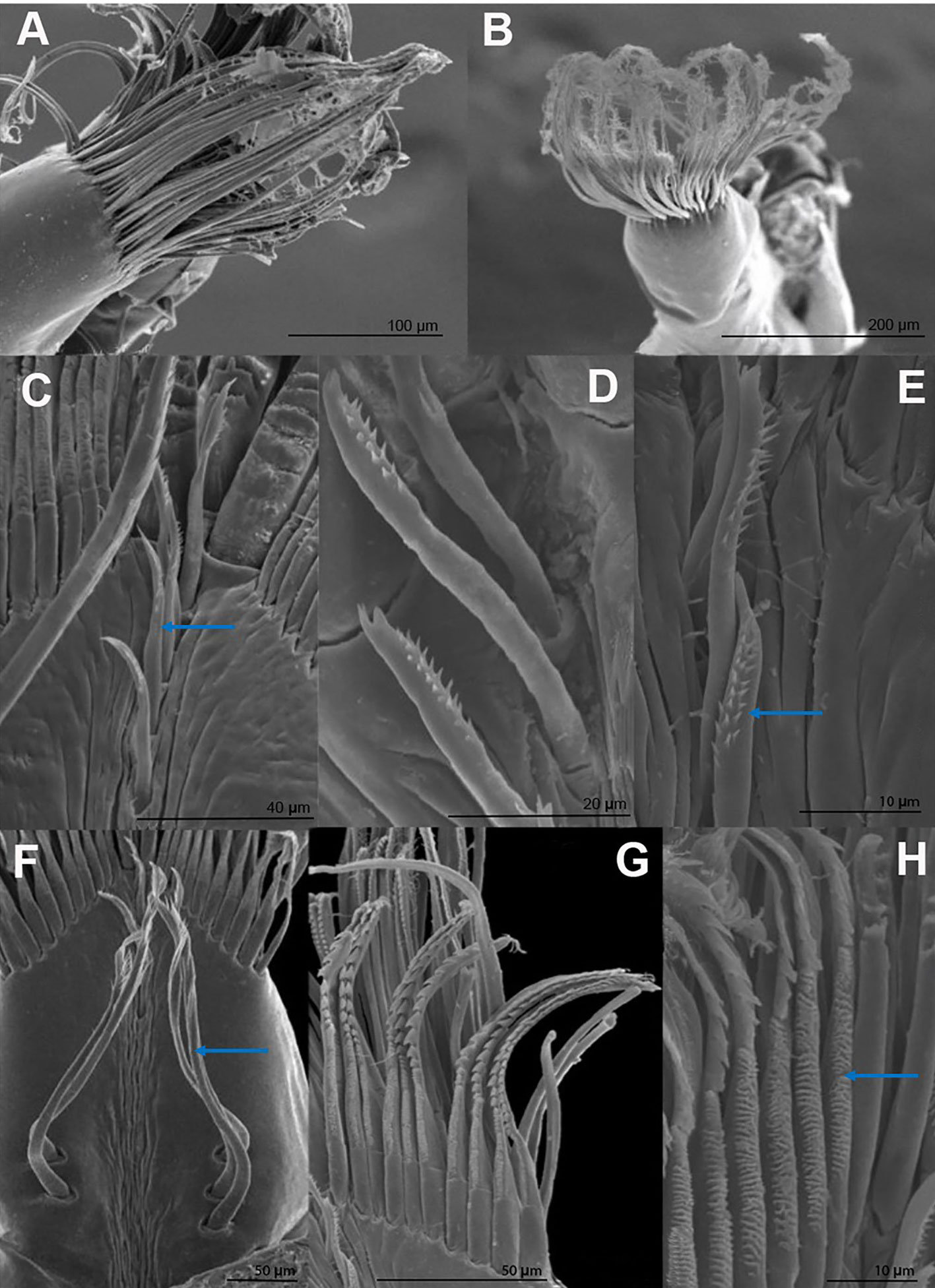
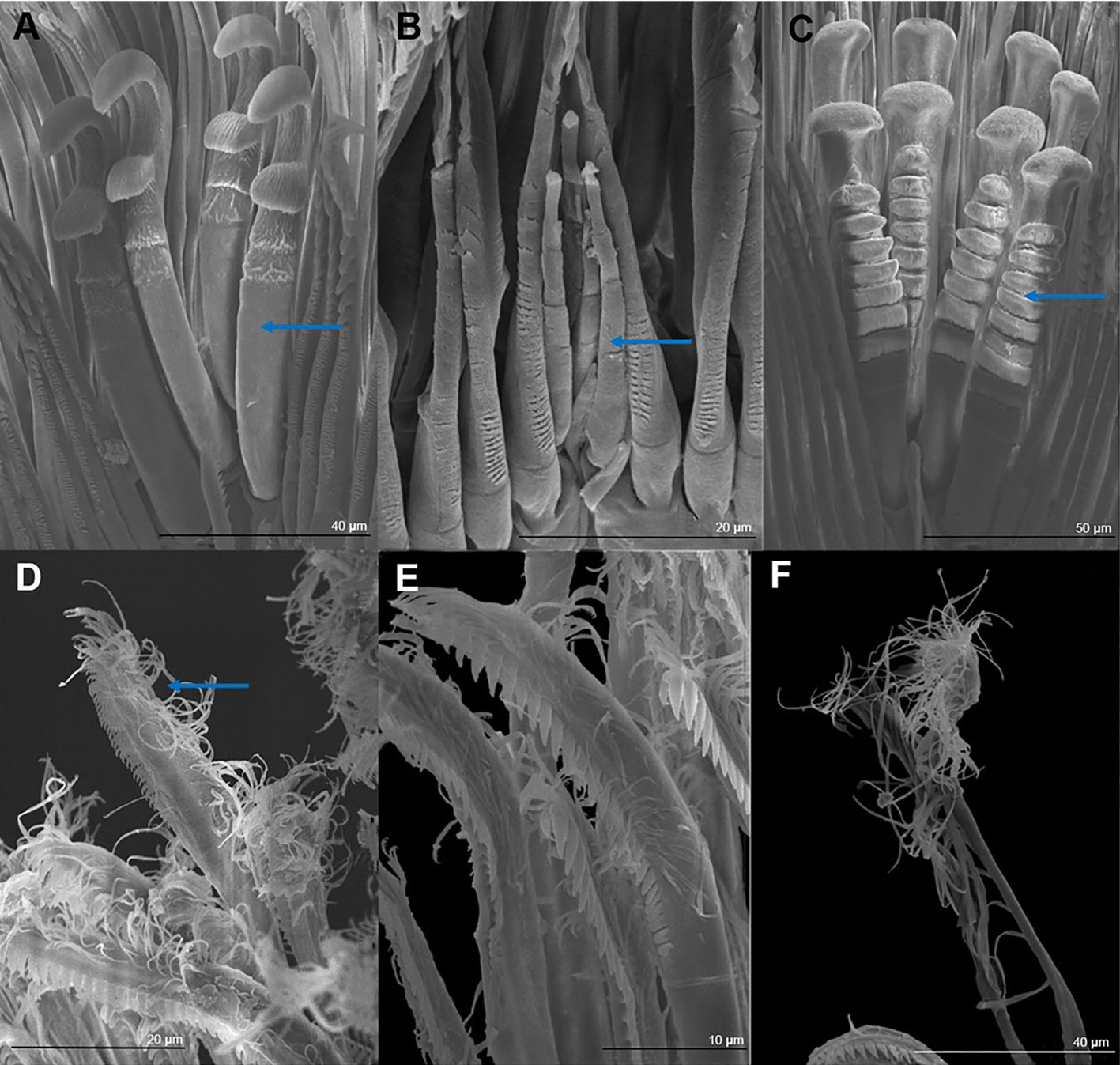
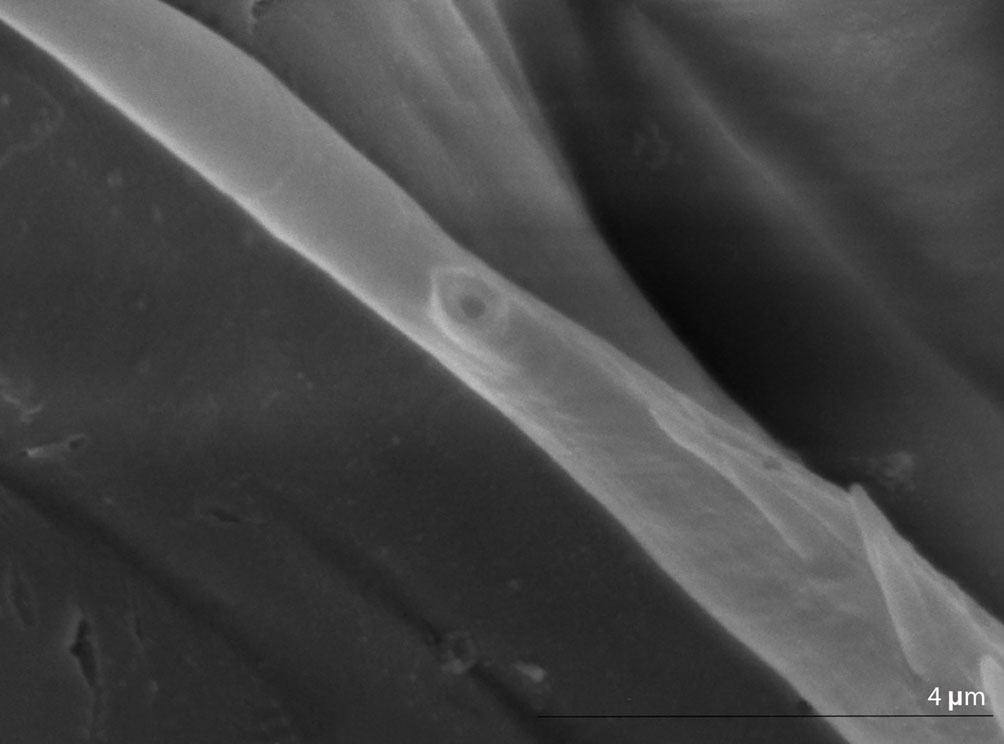
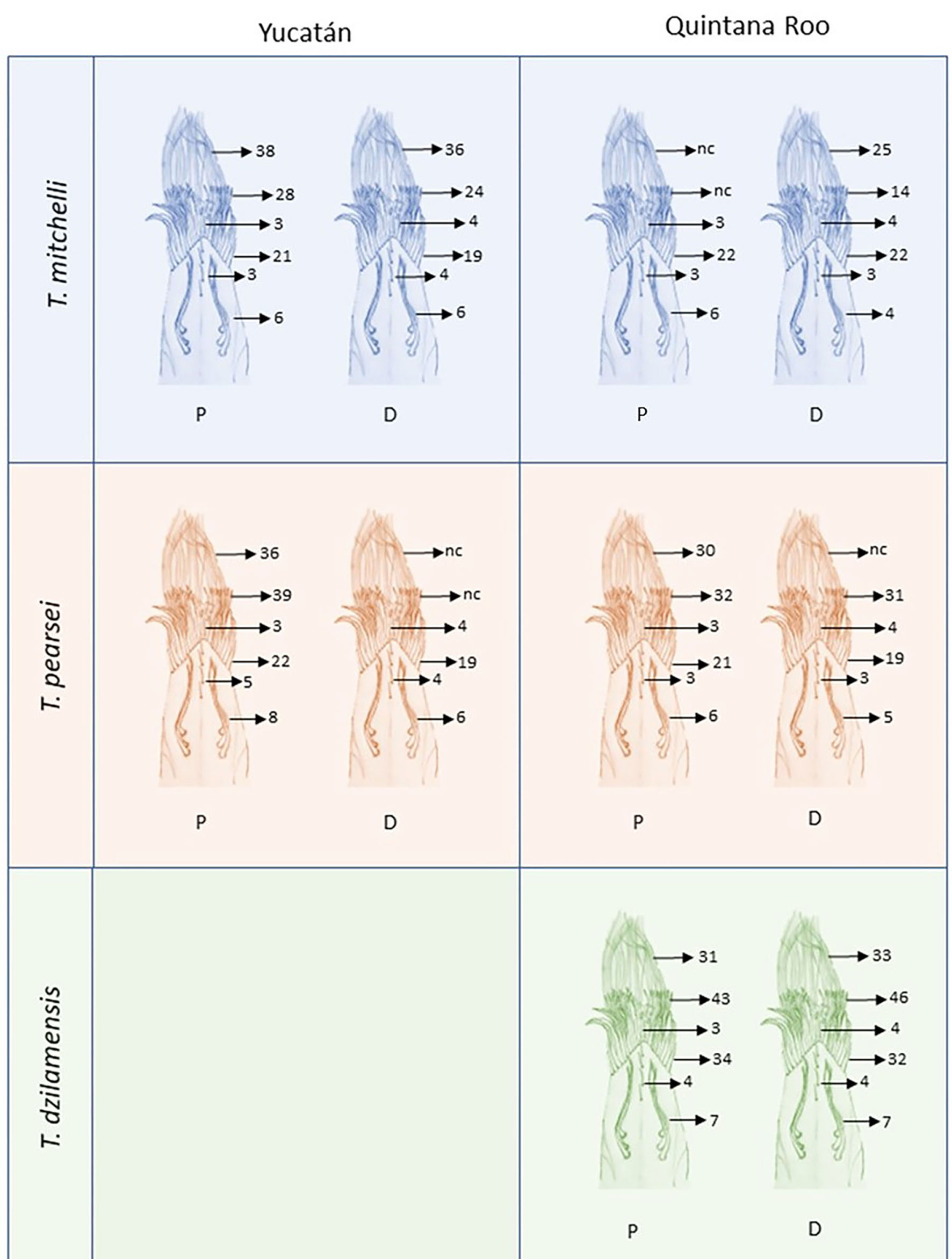
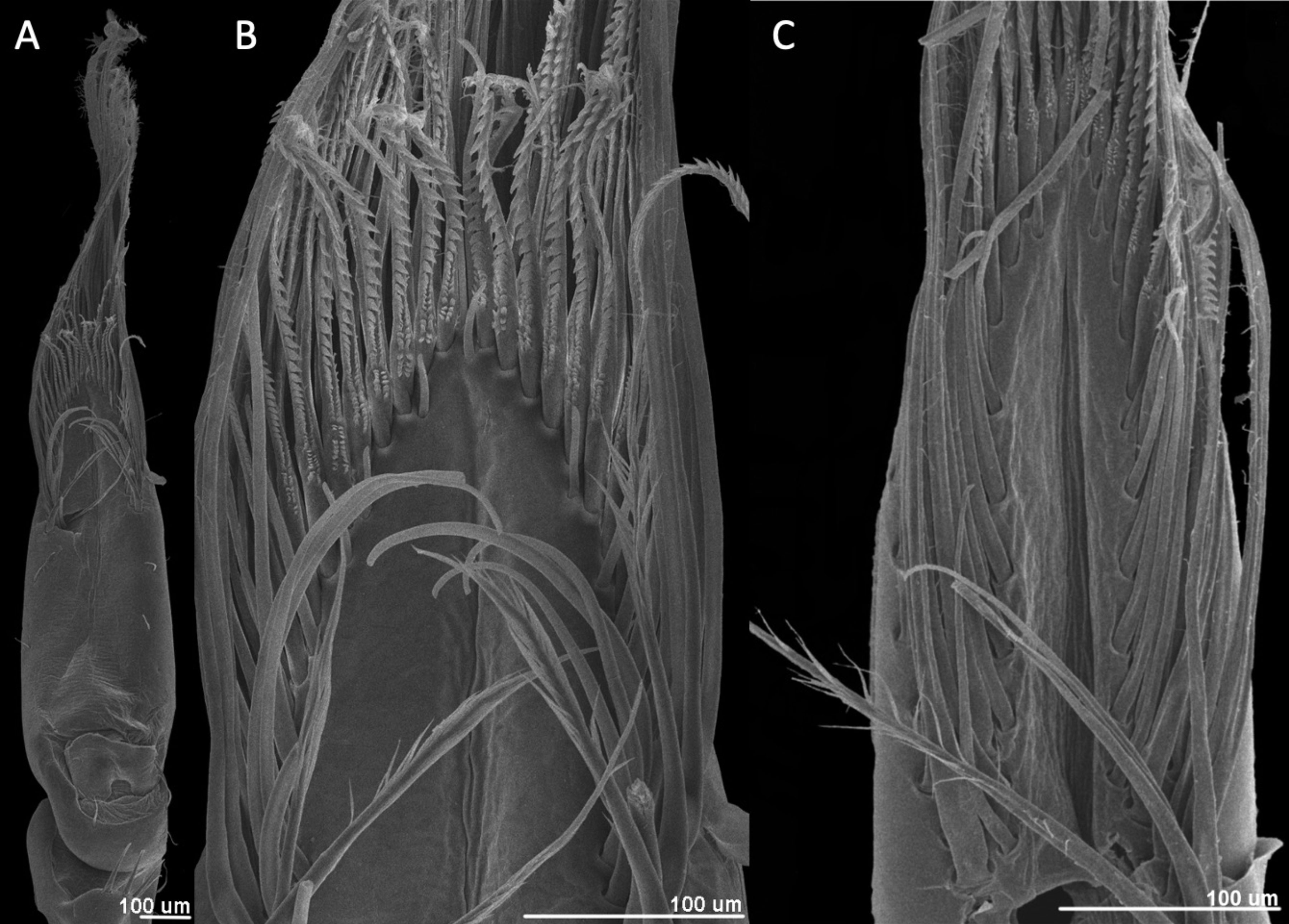
The second-row setae are longer than the first-row ones, slenderer and their distal portion is serrate on their internal surface with simple hair-like projections on the opposite surface in T. mitchelli and T. pearsei, not in T. dzilamensis (Figs. 3, 5D, E). These setae were not measured since there attachment site cannot be observed. They can be classified as papposerrate although the arrangement of setules and denticles is not proximal vs. distal, but rather anterior (serrate) vs. posterior (pappose).
The rear setae in all 3 species are the longest, they can reach 228 mm in T. mitchelli (Fig. 3A; x = 214.8 mm, n = 6), 245 mm in T. pearsei (Fig. 4A; x = 221.5 mm, n = 6) or 385 mm in T. dzilamensis (Fig. 3C; x = 348.1 mm, n = 6). There can be from 25 to 38 in each dactyl or propodus, with some within-species variation too (Fig. 7). These are pappose setae.
In the epigean Potimirim glabra and P. mexicana the setal arrangement is completely different relative to that of Typhlatya. In the species of Potimirim there are no holding, midline, or central setae (Figs. 2, 8). We find 4 different types of setae instead of the 6 types present in Typhlatya. In Potimirim glabra the first row has a continuous row of serrate setae flanked by 8-10 lateral setae proximally. Those lateral setae are long and pappose, and measure about 200 mm long (Fig. 8B). Anterior to the first-row setae appear a row of 4 or 5 inner setae, 30.3 mm long and irregularly spaced (Fig. 8B). The rear setae are pappose and 645 mm in length (Fig. 8A).
Discussion
Fryer (1977) described pappose and papposerrate setae from the chelipeds of Atya innocous and Micratya poeyi and only pappose setae for Atya scabra and Potimirim glabra. Felgenhauer and Abele (1983) reported that the first 2 rows of setae in the pereopods of Atya innocous are chemoreceptors with an apical pore, while the second 2 rows of setae are for scraping and filtering out particles. The midline setae of Typhlatya spp. resemble those described by Felgenhauer and Abele (1983) as they too have an apical pore which suggest a chemoreceptor function. Felgenhauer and Abele (1985) further identified differences between the feeding apparatuses of Atya innocous more adapted to filtering, with more pappose setae, in a lentic environment and Potimirim glabra specialized for scraping, with more serrate setae, in ponds with no flow. Bailey-Brock and Brock (1993) reported a similar feeding apparatus for the related Halocaridina rubra from Hawaii, recognizing serrate, pappose and various types of papposerrate setae in their pereopods. Based on a stable isotope study Burns and Walker (2000), identified that Paratya australiensis fed mainly on filamentous cyanobacteria, which develop biofilms on substrates such as submerged wood. These studies show that research investigating the feeding strategies in atyid shrimps had followed mainly 2 approaches, one based on the functional morphology of feeding appendages and another one that identifies the ingested food items combined with behavioral observations. Atyid shrimps either filter out food particles suspended in the water flow or scrape algal or other plant material from the substrate.
A comparison between epigean atyid species with the hypogean Typhlatya shows that the latter have more setal types in their pereopods, which suggests a higher degree of specialization, possibly related to the nutrient poor cave environment. It could be said, from the functional morphology analysis, that Typhlatya shrimp represent a more specialized scraper. What is known about their diet, derived from stable isotope studies, suggests that these shrimp feed primarily on microbial mats, which in turn incorporate carbon from different sources, and algal or plant fragments mainly from the sediments (Brankovits et al., 2017; Chávez-Solís et al., 2020). Their feeding apparatus is then designed to mainly scrape and trap food particles but could also serve for filter feeding.
The setal arrangement of the cave adapted Typhlatya is quite different from that of the epigean Potimirim. Several important differences are the midline and central setae of Typhlatya are absent in Potimirim, and the rear setae are relatively short in Typhlatya and very long in Potimirim. These differences may reflect the adaptation to different habitats (e.g., an increased number of sensory structures have evolved in stygobitic forms) and consequently the different diet that they have.
The 3 species of Typhlatya studied had basically the same types and arrangement of setae in their pereopods. The observed differences in the number of each setal type could be associated to the size of the individual, since the setae are of approximately the same size in each species; T. dzilamensis is the largest species, and generally T. pearsei is bigger than T. mitchelli.
Two types of setae show morphological variation among species, the central and second row setae. The central setae are robust, thick, hook-like setae. Their function could be to hold to the substrate or to scrape with more strength relative to the first-row setae. A gradient from T. mitchelli to T. pearsei, and T. dzilamensis can be seen in which the number of lamellae on the proximal portion of these setae increases. Another variation was seen in an individual of T. pearsei where the central setae were slender with minute distal hooks (Fig. 5B). As far as we know this is the first time this kind of setae are described. The second-row setae are papposerrate in T. mitchelli and T. pearsei, and serrate in T. dzilamensis. The real scale of variation among species in these setae cannot be now determined due to the reduced number of individuals examined and because it could be species-specific and/or environmentally induced. Future observations may modify the scheme provided here.
Felgenhauer and Abele (1983) identified the first 2 rows of setae in the chelipeds of Atya innocous as chemoreceptors. These setae have 2 longitudinal rows of setules, a distal pore and an apical spur (Felgenhauer & Abele, 1983; Figs. 3G, 7A). These are very similar to the midline seta of Typhlatya spp. (Figs. 4C-E, 6). However, the size of the pore in the seta of Atya innocous is about 15 x 6 mm, whereas in Typhlatya spp. is only about 0.25 mm. In any case, they represent a possible common character in several atyid genera.
As seen in several recent studies (Brankovits et al., 2017; Chávez-Solis et al., 2020; Solis et al., 2023), there is a growing interest in Typhlatya shrimps due to their ecological role in the anchialine food chain and ecosystem. Their importance resides essentially in their relative abundance and particular way of feeding. In a nutrient-poor environment the ability to feed on bacteria could be the one characteristic that have made them successful, and as shown here they possess a feeding apparatus well-adapted for bacterial feeding.
Acknowledgements
We thank Berenit Mendoza Garfias, who operates the scanning electron microscope at the Laboratorio Nacional de la Biodiversidad (LANABIO) of the Instituto de Biología, UNAM for her technical assistance. We gratefully acknowledge the funding received through grants PAPIIT IN206523 (DGAPA-UNAM) and Conacyt Ciencia Básica A1-S-32846. We thank the comments made by Hortencia Obregón and Alejandro Martínez García during the review process.
References
Alvarez, F., Iliffe, T. M., & Villalobos, J. L. (2005). New species of the genus Typhlatya (Decapoda: Atyidae) from anchialine caves in Mexico, the Bahamas, and Honduras. Journal of Crustacean Biology, 25, 81–94. https://doi.org/10.1651/C-2516
Bailey-Brock, J. H., & Brock, R. E. (1993). Feeding, reproduction, and sense organs of the Hawaiian anchialine shrimp Halocaridina rubra (Atyidae). Pacific Science, 47, 338–355.
Beddows, P. A., Smart, P. L., Whitaker, F. F., & Smith, S. L. (2007). Decoupled fresh-saline groundwater circulation of a coastal carbonate aquifer: spatial patterns of temperature and specific electrical conductivity. Journal of Hydrology, 346, 18–32. https://doi.org/10.1016/j.jhydrol.2007.08.013
Brankovits, D., Pohlman, J. W., Niemann, H., Leigh, M. B., Leewis, M. C., Becker, K. W. et al. (2017). Methane- and dissolved organic carbon- fueled microbial loop supports a tropical subterranean estuary ecosystem. Nature Communications, 8, 1835. https://doi.org/10.1038/s41467-017-01776-x
Burns, A., & Walker, K. F. (2000). Biofilms as food for decapods (Atyidae, Palaemonidae) in the River Murray, South Australia. Hydrobiologia, 437, 83–90.
Chávez-Solís, E. M., Solís, C., Simoes, N., & Mascaró, M. (2020). Distribution patterns, carbon sources and niche partitioning in cave shrimps (Atyidae: Typhlatya). Scientific Reports, 10, 12812. https://doi.org/10.1038/s41598-020-69562-2
Felgenhauer, B. E., & Abele, L. G. (1983). Ultrastructure and functional morphology of feeding and associated appendages in the tropical fresh-water shrimp Atya innocuous (Herbst) with notes on its ecology. Journal of Crustacean Biology, 3, 336-363. https://www.jstor.org/stable/1548137
Felgenhauer, B. E., & Abele, L. G. (1985). Feeding structures of two atyid shrimps, with comments on caridean phylogeny. Journal of Crustacean Biology, 5, 397–419. http://www.jstor.org/stable/1547911
Fryer, F. R. S. (1977). Studies on the functional morphology and ecology of the atyid prawns of Dominica. Philosophical Transactions of the Royal Society of London (B), 277, 8–128.
Garm, A. (2004). Revising the definition of the crustacean seta and setal classification systems based on examinations of the mouthpart setae of seven species of decapods. Zoological Journal of the Linnean Society, 142, 233–252.
Garm, A., & Watling, L. (2013). The crustacean integument: setae, setules, and other ornamentation. In L. Watling, & M. Thiel (Eds.), Functional morphology and diversity (pp. 167–198). Oxford: Oxford University Press.
Jurado-Rivera, J. A., Pons, J., Álvarez, F., Botello, A., Humphreys, W. F., Page, T. J. et al. (2017). Phylogenetic evidence that both ancient vicariance and dispersal have contributed to the biogeographic patterns of anchialine cave shrimps. Scientific Reports, 7, 2852. https://doi.org/10.1038/s41598-017-03107-y
Solis, C., Chávez-Solis, E. M., Rodríguez-Ceja, M., Mendez-García, C. G., Ortiz, E., Canto, C. et al. (2023). Linking radiocarbon and trophic webs in karstic groundwater ecosystems in the Yucatan Peninsula, Mexico. Radiocarbon, 64, 1629–1639. https://doi.org/10.1017/RDC.2022.100