Feeding habits define habitat use by bats in an agricultural landscape of the Atlantic Forest
Rafael S. Laurindo a, *, Renan de França Souza b, Guilherme Lemos c, Naim da Silva-Teodoro a, Leonardo Marin-Bonilha a, Fabiana Lucio-de Oliveira c
a Instituto Sul Mineiro de Estudos e Conservação da Natureza, Zona Rural, Caixa Postal 72, Monte Belo/MG, 37115-000, Brazil
b Universidade Salgado de Oliveira, Programa de Pós-Graduação em Zoologia, Manejo e Preservação da Vida Silvestre, Rua Lambari, 10 – Trindade, São Gonçalo/RJ, 24456-570, Brazil
c Instituto Federal do Sul de Minas Gerais, Campus Muzambinho, Departamento de Biologia, Estrada de Muzambinho, Bairro – Morro Preto, Muzambinho/MG, 37890-000, Brazil
*Corresponding author: rafaelslaurindo@gmail.com (R.S. Laurindo)
Received: 28 September 2019; accepted: 17 January 2020
Abstract
Agricultural expansion and fragmentation of native habitats is considered the major cause of declining biodiversity worldwide, negatively impacting the provision and maintenance of nature’s contributions to people. Bats are a key group in agricultural landscapes, playing crucial ecological roles in pollination, seed dispersal and control of invertebrate populations. Due to interspecific differences in diet, morphology, physiology and behavior, bats differ in habitat use, with a species-specific response to landscape changes. In this study, we evaluated how bats from different trophic guilds that can be catched with mist nets relate to different habitats present in a hyper-fragmented agricultural landscape. With the use of mist nets, we captured 309 bats in 30 nights between June 2016 and July 2018. Our results show differences in habitat use between species of different trophic guilds. While insectivorous bats have a positive relationship with the amount of water present at the collection point, frugivorous bats show a negative response. These results reinforce the importance of landscape heterogeneity in agricultural mosaics for maintaining biodiversity.
Keywords: Chiroptera; Landscape ecology; Minas Gerais; Phyllostomidae; Use of space
© 2020 Universidad Nacional Autónoma de México, Instituto de Biología. This is an open access article under the CC BY-NC-ND license
Los hábitos alimenticios definen el uso de hábitat por murciélagos en un paisaje agrícola
Resumen
La expansión agrícola y la consiguiente pérdida y fragmentación de los hábitats nativos se consideran una de las principales causas de la disminución de la biodiversidad en todo el mundo, lo que afecta negativamente la provisión y el mantenimiento de importantes servicios del ecosistema. Los murciélagos representan un grupo clave en los paisajes agrícolas, juegan papeles ecológicos cruciales en la polinización, la dispersión de semillas y el control de las poblaciones de invertebrados. Debido a las diferencias interespecíficas en la dieta, la morfología, la fisiología y el comportamiento, los murciélagos difieren en el uso del hábitat, con respuestas específicas de la especie a los cambios del paisaje. En este estudio evaluamos cómo los murciélagos de diferentes gremios tróficos que pueden ser capturados con redes de niebla, se relacionan con diferentes hábitats presentes en un paisaje agrícola hiperfragmentado. Con la ayuda de redes de niebla, se capturaron 309 murciélagos en 30 noches de recolección entre junio de 2016 y julio de 2018. Nuestros resultados muestran diferencias en el uso del hábitat entre especies de diferentes gremios tróficos. Mientras que los murciélagos insectívoros están positivamente relacionados con la cantidad de agua presente en el punto de recolección, los murciélagos frugívoros muestran una respuesta negativa. Estos resultados refuerzan la importancia de la heterogeneidad del paisaje en los mosaicos agrícolas para mantener la biodiversidad y proporcionar servicios ecosistémicos.
Palabras clave: Chiroptera; Ecología del paisaje; Minas Gerais; Phyllostomidae; Uso del espacio
© 2020 Universidad Nacional Autónoma de México, Instituto de Biología. Este es un artículo Open Access bajo la licencia CC BY-NC-ND
Introduction
The conversion of forest into agricultural landscapes is one of the main threats to biodiversity worldwide, especially in tropical environments (Lambin et al., 2001). It is a powerful driver to local extinctions, changing composition and abundance of fauna assemblages (Green, 2005). Although the negative effects of habitat loss are known, environmental exploitation and deforestation continues to be a trend due to the growth of human populations and the expansion of agriculture (DeFries et al., 2010). On the other hand, applied environmental research has provided reasonable data in order to mitigate the loss of biodiversity and maintain nature’s contributions to people (Landis, 2017). When management includes structural heterogeneity and species composition in agricultural landscapes, it is possible to find patterns of assemblages and to try to restore ecosystem functions previously lost (Duru et al., 2015; Fahrig et al., 2011).
Chiroptera is diversified with respect to both, species richness and functional ecology. In tropical environments, bats play essential ecological roles owing to their mobility and use of space, and also their efficiency in utilizing different food sources and roosts (Denzinger & Schnitzler, 2013; Ferreira et al., 2017). This determines how bat assemblages relate to agricultural landscapes, and their leading roles in seed dispersion, pollination, and control of insect population in these areas (Voigt & Kingston, 2016). The response of bat assemblages to changes in the landscape is species- or ensemble-specific (Montaño-Centellas et al., 2015; Pinto & Keitt, 2008) due to their diets, morphology, physiology and behavior (Denzinger & Schnitzler, 2013; Heer et al., 2015). The morphology of bat wings is considered to be a good proxy for the type of diet and the habitat use associated with each species (Marinello & Bernard, 2014). Aerial insectivores have wings adapted for fast flight and low maneuverability, ideal for open environments with few obstructions. On the other hand, the shape of the wings of frugivores indicate slower flight and high maneuverability, ideal for flying inside forests (Marinello & Bernard, 2014). The availability and distribution of food resources in the landscape also influences bat activity and habitat use. Frugivorous and nectarivorous species are common in edges and fragments of forests, as well as preserved areas, where fruits are more abundant (Medina et al., 2007; Williams-Guillén & Perfecto, 2010), while insectivores are commonly recorded foraging near to waterbodies and open areas (Costa et al., 2012).
Research on neotropical bat assemblages in agricultural landscapes is necessary not only to understand population dynamics, but also to find patterns on their long-term persistence in human-modified areas and their association with the landscape structure. These data are fundamental to foster better strategies for ecological management and maintenance or restoration of nature’s contributions to people (Smith & Sullivan, 2014).
Previous studies have demonstrated that composition and configuration of agricultural landscapes affect the diversity and the activity of different bat species (Heim et al., 2017; Lentini et al., 2012). This is because species from different trophic guilds differ in their preference for using microhabitats in agricultural landscapes (Heim et al., 2017). We have therefore sampled bats in 10 locations with different landscape composition (i.e., forest cover, agricultural matrix, presence of waterbodies), in order to assess the species-environment relationship. We expected: 1) a higher number of insectivorous bats captured in areas with higher waterbody coverage (Costa et al., 2012) and areas with urban constructions, since many species use these buildings as roost; 2) frugivorous and nectarivorous species would be captured more frequently in areas with higher forest cover (Muylaert et al., 2016).
Materials and methods
We sampled an agricultural landscape formed by different types of agricultural matrices (i.e., coffee, eucalyptus monoculture, pasture and small forest remnants), in the municipality of Muzambinho, state of Minas Gerais, in southeastern Brazil. This region is classified as an ecotone between 2 biomes that are considered to be biodiversity hotspots: Atlantic Forest and Cerrado (Weyland-Vieira et al., 2014). It is also considered to be part of the Atlantic Plateau, an area of highlands at an elevation varying between 800-1,200 m. The climate in this landscape is classified as mild sub-humid mesothermal, with an average annual temperature of 18 ºC. However, during the coldest months, the minimum daily temperature can vary between 0 and -4 °C, mainly due to the elevation.
We used images provided by the National Institute for Space Research (INPE). For the composition of false color RGB (red-green-blue) in mapping, we used the sequence B03 (R), B04 (G) and B02 (B), making it possible to identify and interpret the features shown in the image, with well-defined boundaries between soil and water, as well as a reliable characterization of the area with vegetation cover. We determined types of land use by applying the supervised classification method when the image was to be attributed to pre-fixed interest classes. We employed a maximum likelihood algorithm (MaxVer). This is one of the most common supervised classification methods, which takes into account the weighting of the distances between the averages of the digital levels of the classes and the pixel, using statistical parameters, considering the normal probability distribution for each class (Facco et al., 2017).
The mapping classes were defined based on a detailed preview of the images, supported by the Google Earth software, which has updated images from the study period. For this study, we defined the following classes of land use: agriculture, water, urbanized area, native forest, and planted forest. The class “water” comprises any waterbody located at the collection spot, like dams, lakes and rivers. We then created a shapefile vector file for sampling points, with circular buffers of 100 m radius around each one, and quantified, in hectares, the area of each land use class in the buffer.
The reliability of the digital classification was estimated from the confusion matrix of the training area samples, obtaining a global accuracy index of 92.72% and a Kappa index of 0.8949 (89.49%) for the classified image. All analyses were performed using ArcGIS version 10.2 and ENVI version 5.0 software.
We carried out fieldwork on 10 sampling points (Fig. 1) from June 2016 to July 2018. Each area was sampled for 3 non-consecutive nights, 1 night in the dry season and 2 nights in the rainy one. In order to avoid seasonal variations, in each one of the 3 sampling campaigns all 10 spots were sampled within a maximum interval of 2 months. To capture bats, we used 7 mist nets (9 × 3 m, 25 mm mesh) that were set at ground level and remained open for 6 h each evening, from sunset. Captured bats were weighed and the length of their forearm was measured. We also noted down the sex and classified the bats in general age categories (juvenile or adult) based on the level of epiphysis ossification in the arm, metacarpals and phalanxes, according to Anthony (1988). We identified species during the fieldwork using descriptions available in the literature (Dias & Peracchi, 2008; Reis et al., 2007). Bats were classified into trophic guilds as suggested by Kalko et al. (1996). Fieldwork protocols followed the guidelines of the American Society of Mammalogists (Sikes et al., 2016). Some specimens were euthanized for taxonomic confirmation and added to the Mammal Collection of the Federal University of Lavras (Appendix 1). This study was approved by Instituto Brasileiro do Meio Ambiente – IBAMA (SISBio, license number 40325).
We tested our results for prediction of the dependent variables (insectivores, and frugivores + nectarivores) by taking agriculture, native forest, planted forest, urbanization and water as predictors. First, we assessed the normal distribution of these predictors using the Shapiro-Wilk test. Collinearity between the predictor variables was tested by calculating Spearman’s rank correlation and setting the threshold for acceptable values at 0.5-0.7. Since water was highly correlated (> 0.8) with native forest and urbanization (Appendix 2), we constructed separated models for the dependent variables considering water as the only predictor. We also developed 2 global models, one with insectivores as a dependent variable and the other with frugivores + nectarivores as a dependent variable, and agriculture, native forest, planted forest and urbanization as predictors. In order to achieve the best model addressing overdispersion, given that the dependent variables are count data, we tested the global models with Poisson, Quasipoisson and Negative Binomial dispersions. For frugivore + nectarivore, Negative Binomial was the most suitable dispersion and we considered it as the global model. Conversely, for insectivores the most suitable dispersion was Poisson, since the Negative Binomial model showed an inflated dispersion parameter (theta) and the iterations were not precisely computed. We also tested our data for linear relationships between predictors and dependent variables in the 2 mentioned global models based on component plus residual plots (Fox & Weisberg, 2019). The predictor land use classes agriculture and planted forest were transformed by their square root in the final Negative Binomial global model, in order to achieve linearity.
Finally, the 2 global models (insectivores and frugivore + nectarivore) were used to carry out model selection following the Akaike second-order information criterion (AICc) (Burnham & Anderson, 2002). We took for best model the one with the lowest value for AICc (Burnham & Anderson, 2002). However, in order to benefit from gathering important information for other well fitted and equally parsimonious models, we computed the average of the models selected under delta < 4 (Burnham, et al., 2011; Symonds & Moussalli, 2011 …no aparece en las Referencias). The outcome of the model average was used to describe the effects of the predictors selected in those models (delta < 4) in the response variables. All analyses were performed using the software R version 3.6.1, with the packages “PerformanceAnalytics”, “car”, and “MuMIn”.
Results
We captured 309 bats of 21 species on 3 families: Phyllostomidae (9 species), Molossidae (5 species) and Vespertilionidae (7 species). Phyllostomidae bats were the most abundant, with 238 bats captured, 77% of all captures (Table 1). The most abundant species in the study area were Sturnira lilium (n = 124), Artibeus lituratus (n = 36) and Carollia perspicillata (n = 25). Among Vespertilionidae, Myotis albescens and M. nigricans were the most abundant, with n = 20 (6.47% of the total), and n = 18 (5.83% of the total), respectively (Table 1).

Table 1
Captures (N) and percentage of total capture (%) by bat species in an agricultural landscape in the municipality of Muzambinho, Minas Gerais, Brazil.
Family | N | % |
Phyllostomidae | ||
Carollia perspicillata (Linnaeus, 1758) | 25 | 8.09 |
Anoura geoffroyi Gray, 1838 | 4 | 1.29 |
Anoura caudifer (É. Geoffroy, 1818) | 18 | 5.84 |
Artibeus lituratus (Olfers, 1818) | 36 | 11.65 |
Artibeus planirostris (Spix, 1823) | 3 | 0.97 |
Platyrrhinus lineatus (E. Geoffroy, 1810) | 24 | 7.77 |
Sturnira lilium (E. Geoffroy, 1810) | 124 | 40.13 |
Phyllostomus hastatus (Pallas, 1767) | 1 | 0.32 |
Vampyressa pusilla (Wagner, 1843) | 3 | 0.97 |
Vespertilionidae | ||
Myotis albescens (É. Geoffroy, 1806) | 20 | 6.47 |
Myotis ruber (E. Geoffroy, 1806) | 2 | 0.65 |
Myotis riparius Handley, 1960 | 15 | 4.85 |
Myotis lavali (Moratelli, Peracchi, Dias & Oliveira, 2011) | 2 | 0.32 |
Myotis nigricans (Schinz, 1821) | 18 | 5.84 |
Histiotus velatus (I. Geoffroy, 1824) | 2 | 0.97 |
Eptesicus furinalis (d’Orbigny, 1847) | 2 | 0.65 |
Molossidae | ||
Molossus molossus (Pallas, 1766) | 3 | 0.97 |
Molossus aztecus Saussure, 1860 | 1 | 0.32 |
Nyctinomops laticaudatus (E. Geoffroy, 1805) | 1 | 0.32 |
Molossops neglectus Williams & Genoways, 1980 | 4 | 1.29 |
Eumops perotis (Schinz, 1821) | 1 | 0.32 |
Total | 309 | 100 |
To evaluate the effect of the landscape on the number of insectivorous bat catches, 3 models with ΔAICc < 4 (Table 2) were selected. All land use types evaluated in this study had a significant influence over the response variable (Tables 3, 4). While the number of capture of insectivorous bats had a positive correlation with water-covered area, planted forest and urbanization, the correlation was negative with increased native forest cover and agricultural areas (Fig. 2A).
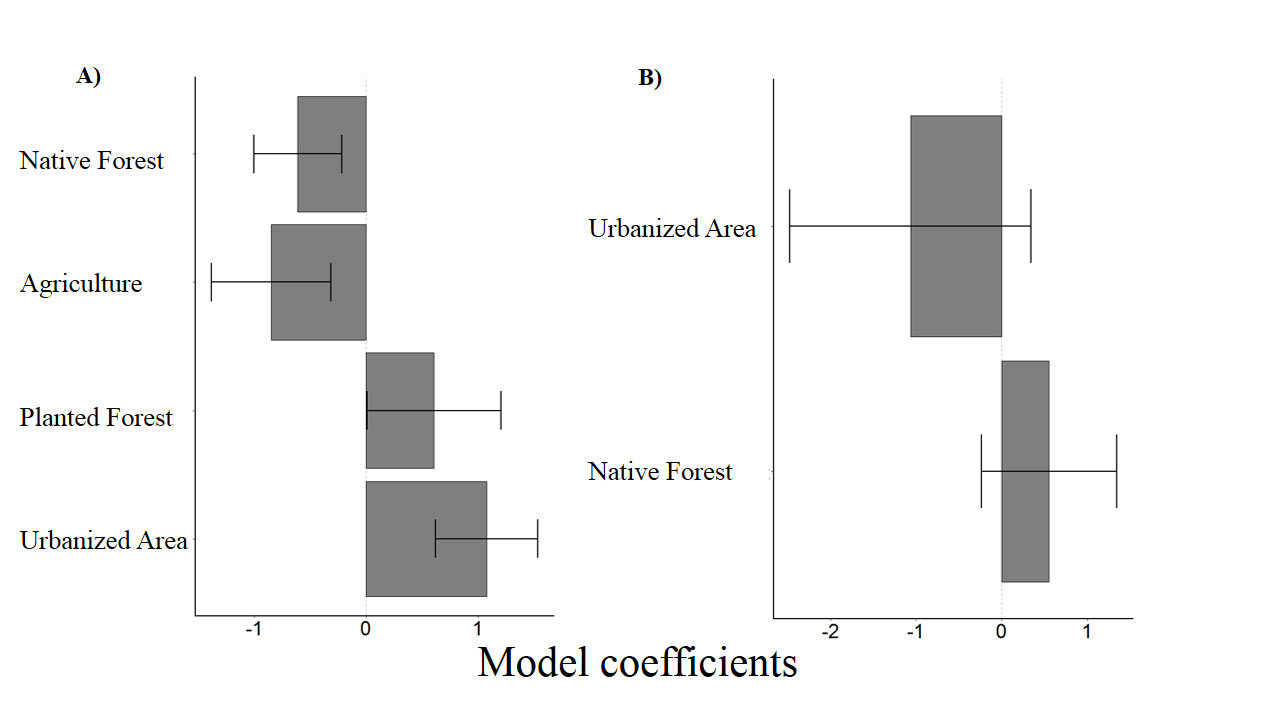
Table 2
Ranking of the best linear models to predict bat capture as a function of landscape structure. Models were selected according to the second-order Akaike Information Criterion (AICc) corrected for small samples.
Response variable (bat abundance) | Models | AICc | ΔAICc | Wi |
Insectivores | Agriculture + native forest | 61.0 | 0.00 | 0.623 |
Planted forest + urbanization | 64.2 | 3.17 | 0.128 | |
Urbanization | 65.0 | 3.95 | 0.087 | |
Frugivores and nectarivores | Null Model | 89.4 | 0.00 | 0.467 |
Urbanization | 91.3 | 1.97 | 0.175 | |
Native forest | 91.3 | 2.00 | 0.175 |
For frugivorous and nectarivorous bats, 3 models with ΔAICc < 4 (Table 2) were selected, but none of the explanatory variables (forest and urbanization) present in the best models had a significant effect that could explain the number of capture (Table 3; Fig. 2B). On the other hand, our results demonstrate a negative correlation between area covered by waterbodies and the number of frugivorous and nectarivorous bats (Table 4; Fig. 3).
Table 3
Model-averaged parameters of the best linear models to predict capture of insectivorous and frugivorous + nectarivorous bats as a function of landscape structure. β indicates slopes and reflect the intensity and direction of the association between predictor and response variables
Response variable (bat abundance) | Predictive variable | β | Z-value | p |
Insectivores | Agriculture | -0.84 | 3.12 | 0.001 |
Native forest | -0.61 | 3.05 | 0.002 | |
Planted forest | 0.60 | 1.98 | 0.04 | |
Urbanization | 1.07 | 4.61 | 0.001 | |
Frugivores and nectarivores | Urbanization | -1.06 | 1.48 | 0.13 |
Native forest | 0.55 | 1.37 | 0.17 |
Table 4
Influence of the area covered by waterbodies on the number of capture of frugivorous + nectarivorous and insectivorous bats. β indicates slopes and reflects the intensity and the direction of the correlation between predictor and response variables.
Response variable (bat abundance) | Predictive variable | β | Z-value | p |
Insectivores | Water | 1.46 | 2.57 | 0.01 |
Frugivores and nectarivores | Water | -1.62 | -2.28 | 0.02 |

Discussion
According to our predictions, the land use composition found in each sampling site affects differently the number of catches of frugivorous, nectarivorous and insectivorous bats, due to the morphological, physiological or nutritional requirements of each species (Denzinger & Schnitzler, 2013; Heim et al., 2015). The capture of insectivorous bats revealed significant correlations, both positive and negative, with all types of land use considered in this study. On the other hand, contrary to our predictions, the capture of frugivorous and nectarivorous bats revealed only a significant and negative correlation to an increase in the area covered by waterbodies at the sampling spots.
For insectivorous bats, we found a significant and positive relationship between the area covered by waterbodies in the buffer and the abundance of insectivorous bats. The high activity of insectivorous bats in both natural and anthropogenic aquatic ecosystems is well documented (Costa et al., 2012; López-González et al., 2015).
Bats use these foraging habitats due to the high abundance of insects and the absence of obstacles, as many species have a fast and non-maneuverable flight (Fukui et al., 2006; Hagen & Sabo, 2014). Recent research highlights the importance not only of maintaining native vegetation in agricultural landscapes, but also of adding water sources, such as artificial weirs, which may help control insect pests due to the high activity of insectivorous bats near these environments (Park, 2015).
Our results also show a positive correlation between the percentage of planted forest (Eucalyptus sp.) and the number of captures of insectivorous bats. Planted forests (monocultures) constitute a simplification of the structure and the composition of the vegetation (Lopes et al., 2015), imposing less restrictions to the flight and the orientation of insectivorous bats. Planted forests can be used during relocation or as a foraging place (Barros et al., 2014). Urbanization has also positively influenced the number of captures, because many species of insectivorous bats use buildings as shelter (Jung & Threlfall, 2018). The presence of artificial lights in buildings can also attract various bat species, due to the abundance of insects in these areas (Jung & Kalko, 2011), thus influencing the number of captures.
On the other hand, the increase in native vegetation cover negatively influenced the number of captures. All forest fragments present in the study area are small, which makes them more susceptible to the edge effect (Ewers et al., 2007). Changes in vegetation structure at fragment edges, such as higher plant density and dense understory, may impose flight restrictions on many insectivorous bat species, causing them to avoid these locations (Suárez-Rubio et al., 2018). The increase in agricultural areas has also negatively influenced the capture of insectivorous bats. The intensification of agriculture may lead to a decrease in the abundance and the diversity of nocturnal insects that are part of the diet of insectivorous bats, resulting in a lower foraging activity in these environments (Wickramasinghe et al., 2004). Furthermore, pesticide use in agricultural crops is also related to episodes of bat mortality around the world, which may cause reduced diversity in these landscapes (O’Shea et al., 2016).
For frugivorous bats, only the area covered by waterbodies at the sampling point significantly influenced negatively the abundance. Most points with water correspond to small dams without riparian vegetation, where frugivorous bats generally have reduced activity when in open areas, due to the higher risk of predation (Ripperger et al., 2015). Contrary to our prediction, we did not observe a significant correlation between area covered of native vegetation and abundance of frugivorous bats. Many species of frugivorous bats are capable of flying long distances (Trevelin et al., 2013) and are, to some extent, tolerant of changes in land use (Gonçalves et al., 2017), which may allow them to use the entire landscape for foraging.
Briefly, we have demonstrated that on a local scale, frugivorous and insectivorous bat species differ in habitat use in agricultural landscapes, corroborating other studies, and suggesting a species- or guild-specific response to different components in the landscape (Klingbeil & Willig, 2009). Although our study has revealed a significantly higher number of catches of insectivorous bats, when compared to other studies carried out in nearby locations where the Phyllostomidae family represents around 90% of the richness our results need to be interpreted with caution, as we have only used mist nets for capturing bats, which may underestimate the abundance and richness of insectivorous bats, as many species can easily detect and avoid mist nets or fly above them (O’Farrell & Gannon, 1999; Silva & Bernard, 2017). Lastly, it is important to highlight that the region where the study was carried out is highly fragmented, and the composition of the bat community may reflect the history of changes and land use and the absence of large forest fragments, since we have not captured any species of gleaning insectivores, which are more sensitive to forest loss (Meyer et al., 2007).
Acknowledgements
To the Federal Institute of Southern Minas (Muzambinho campus) for having allowed us to conduct this study in the areas they manage and for having provided us with logistical support. NST and GL thank the Foundation for Research Support in the State of Minas Gerais (FAPEMIG) for having granted them the PIBIC scholarships for scientific initiation. We are also grateful to Écio Diniz for his support with the statistical analysis, and to Anna Civolani for proofreading. We extend our gratitude to the peer reviewers for the contributions they have made to this manuscript.
Appendix 1. Voucher numbers of catalogued specimens at the Mammal Collection of the Federal University of Lavras.
Eptesicus furinalis CMUFLA 2484 and 3114, Histiotus velatus CMUFLA 2782 and 3116, Molossops neglectus CMUFLA 3142, 3143 and 3156, Molossus aztecus CMUFLA 2847, Molossus molossus CMUFLA 3115, Myotis albescens CMUFLA 2488 and 2489, Myotis lavali CMUFLA 2917 and 2918, Myotis nigricans CMUFLA 2485 and 2487, Myotis ruber CMUFLA 2486, Nyctinomops laticaudatus CMUFLA 2482
Appendix 2. Correlations between predictor variables (classes of land use).
References
Anthony, E. L. P. (1988). Age determination in bats. In T. H. Kunz (Ed.), Ecological and behavioral methods for the study of bats (pp. 47–58). Washington D.C.: Smithsonian Institution Press.
Barros, M. A. S, Pessoa, D. M. A., & Rui, A. M. (2014). Habitat use and seasonal activity of insectivorous bats (Mammalia: Chiroptera) in the grasslands of southern Brazil. Zoologia (Curitiba), 31, 153–161. http://dx.doi.org/10.1590/S1984-46702014000200006
Burnham, K. P., & Anderson, D. R. (2002). Model selection and multimodel inference: A practical information theoretic approach. New York: Springer.
Burnham, K. P., Anderson, D. R., & Huyvaert, K. P. (2011) AIC model selection and multimodel inference in behavioral ecology: Some background, observations, and comparisons. Behavioral Ecology and Sociobiology, 65, 23–35. https://doi.org/10.1007/s00265-010-1029-6
Costa, L. M., Luz, J. L., & Esbérard, C. E. L. (2012). Riqueza de morcegos insetívoros em lagoas no Estado do Rio de Janeiro, Brasil. Papéis Avulsos de Zoologia, 52, 7–19. https://doi.org/10.1590/s0031-10492012000200001
DeFries, R. S., Rudel, T., Uriarte, M., & Hansen, M. (2010). Deforestation driven by urban population growth and agricultural trade in the twenty-first century. Nature Geoscience, 3, 178–181. https://doi.org/10.1038/ngeo756
Denzinger, A., & Schnitzler, H. U. (2013). Bat guilds, a concept to classify the highly diverse foraging and echolocation behaviors of microchiropteran bats. Frontiers in Physiology, 4, 164. https://doi.org/10.3389/fphys.2013.00164
Dias, D., & Peracchi, A. L. (2008). Quirópteros da Reserva Biológica do Tinguá, estado do Rio de Janeiro, sudeste do Brasil (Mammalia: Chiroptera). Revista Brasileira de Zoologia, 25, 333–369. https://doi.org/10.1590/s0101-81752008000200023
Duru, M., Therond, O., Martin, G., Martin-Clouaire, R., Magne, M. A., Justes, E. et al. (2015). How to implement biodiversity-based agriculture to enhance ecosystem services: a review. Agronomy for Sustainable Development, 35, 1259–1281. https://doi.org/10.1007/s13593-015-0306-1
Ewers, R. M., Thorpe, S., & Didham, R. K. (2007). Synergistic interactions between edge and area effects in a heavily fragmented landscape. Ecology, 88, 96–106. https://doi.org/10.1890/0012-9658(2007)88[96:sibeaa]2.0.co;2
Facco, D. S., Benedetti, A. C., Kaiser, E. A., & Filho, W. P. (2017). Avaliação da dinâmica do uso e cobertura da terra no município de Faxinal do Soturno no estado do Rio Grande do Sul. In A. Perez-Filho, & R. R. Amorim (Eds.), Os desafios da Geografia Física na fronteira do conhecimento (pp. 6846–6855). Campinas: UNICAMP. https://doi.org/10.20396/sbgfa.v1i2017.1876
Fahrig, L., Baudry, J., Brotons, L., Burel, F. G., Crist, T. O., Fuller, R. J. et al. (2011). Functional landscape heterogeneity and animal biodiversity in agricultural landscapes: heterogeneity and biodiversity. Ecology Letters, 14, 101–112. https://doi.org/10.1111/j.1461-0248.2010.01559.x
Ferreira, D. F., Rocha, R., López-Baucells, A., Farneda, F. Z., Carreiras, J. M. B., Palmeirim, J. M. et al. (2017). Season-modulated responses of neotropical bats to forest fragmentation. Ecology and Evolution, 7, 4059–4071. https://doi.org/10.1002/ece3.3005
Fox, J., & Weisberg, S. (2019). An R companion to applied regression, 3. Ed. Thousand Oaks: Sage.
Fukui, D., Murakami, M., Nakano, S., & Aoi, T. (2006). Effect of emergent aquatic insects on bat foraging in a riparian forest. Journal of Animal Ecology, 75, 1252–1258. https://doi.org/10.1111/j.1365-2656.2006.01146.x
Gonçalves, F., Fischer, E., & Dirzo, R. (2017). Forest conversion to cattle ranching differentially affects taxonomic and functional groups of Neotropical bats. Biological Conservation, 210, 343–348. https://doi.org/10.1016/j.biocon.2017.04.021
Green, R. E. (2005). Farming and the fate of wild nature. Science, 307, 550–555. https://doi.org/10.1126/science.1106049
Hagen, E. M., & Sabo, J. L. (2014). Temporal variability in insectivorous bat activity along two desert streams with contrasting patterns of prey availability. Journal of Arid Environments, 102, 104-112. https://doi.org/10.1016/j.jaridenv.2013.11.016
Heer, K., Helbig-Bonitz, M., Fernandes, R. G., Mello, M. A. R., & Kalko, E. K. V. (2015). Effects of land use on bat diversity in a complex plantation–forest landscape in northeastern Brazil. Journal of Mammalogy, 96, 720–731. https://doi.org/10.1093/jmammal/gyv068
Heim, O., Treitler, J. T., Tschapka, M., Knörnschild, M., & Jung, K. (2015). The importance of landscape elements for bat activity and species richness in agricultural areas. Plos One, 10, e0134443. https://doi.org/10.1371/journal.pone.0134443
Heim, O., Lorenz, L., Kramer-Schadt, S., Jung, K., Voigt, C. C., & Eccard, J. A. (2017). Landscape and scale-dependent spatial niches of bats foraging above intensively used arable fields. Ecological Processes, 6, 1–15. https://doi.org/10.1186/s13717-017-0091-7
Jung, K., & Kalko, E. K. (2011). Adaptability and vulnerability of high flying Neotropical aerial insectivorous bats to urbanization. Diversity and Distributions, 17, 262–274. https://doi.org/10.1111/j.1472-4642.2010.00738.x
Jung, K., & Threlfall, C. G. (2018). Trait-dependent tolerance of bats to urbanization: a global meta-analysis. Proceedings of the Royal Society B: Biological Sciences, 285, 20181222. https://doi.org/10.1098/rspb.2018.1222
Kalko, E. K., Handley, C. O. Jr., & Handley, D. (1996). Organization, diversity, and long-term dynamics of a Neotropical bat community. In M. L. Cody, & J. A. Smallwood (Eds.), Long-term studies of vertebrate communities (pp. 503–553). Academic Press. https://doi.org/10.1016/b978-012178075-3/50017-9
Klingbeil, B. T., & Willig, M. R. (2009). Guild-specific responses of bats to landscape composition and configuration in fragmented Amazonian rainforest. Journal of Applied Ecology, 46, 203–213. https://doi.org/10.1111/j.1365-2664.2008.01594.x
Lambin, E. F., Turner, B. L., Geist, H. J., Agbola, B., Angelsen, A., Bruce, J. et al. (2001). The causes of land-use and land-cover change: moving beyond the myths. Global Environmental Change, 11, 261–269.
Landis, D. A. (2017). Designing agricultural landscapes for biodiversity-based ecosystem services. Basic and Applied Ecology, 18, 1–12. https://doi.org/10.1016/j.baae.2016.07.005
Lentini, P. E., Gibbons, P., Fischer, J., Law, B., Hanspach, J., & Martin, T. G. (2012). Bats in a farming landscape benefit from linear remnants and unimproved pastures. Plos One, 7, e48201. https://doi.org/10.1371/journal.pone.0048201
Lopes, I. T., Gussoni, C. O., Demarchi, L. O., De Almeida, A., & Pizo, M. A. (2015). Diversity of understory birds in old stands of native and eucalyptus plantations. Restoration Ecology, 23, 662–669. https://doi.org/10.1111/rec.12216
López-González, C., Gómez-Ruiz, E. P., Lozano, A., & López-Wilchis, R. (2015). Activity of insectivorous bats associated with cattle ponds at La Michilía Biosphere Reserve, Durango, Mexico: implications for conservation. Acta Chiropterologica, 17, 117–129. https://doi.org/10.3161/15081109acc2015.17.1.010
Marinello, M. M., & Bernard, E. (2014). Wing morphology of neotropical bats: a quantitative and qualitative analysis with implications for habitat use. Canadian Journal of Zoology, 92, 141–147. https://doi.org/10.1139/cjz-2013-0127
Medina, A., Harvey, C. A., Merlo, D. S., Vílchez, S., & Hernández, B. (2007). Bat diversity and movement in an agricultural landscape in Matiguás, Nicaragua. Biotropica, 39, 120–128. https://doi.org/10.1111/j.1744-7429.2006.00240.x
Meyer, C. F. J, Fründ, J., Lizano, W. P., & Kalko, E. K. V. (2007). Ecological correlates of vulnerability to fragmentation in Neotropical bats: Correlates of fragmentation sensitivity in Neotropical bats. Journal of Applied Ecology, 45, 381–391. https://doi.org/10.1111/j.1365-2664.2007.01389.x
Montaño-Centellas, F., Moya, M. I., Aguirre, L. F., Galeón, R., Palabral, O., Hurtado, R. et al. (2015). Community and species-level responses of phyllostomid bats to a disturbance gradient in the tropical Andes. Acta Oecologica, 62, 10–17. https://doi.org/10.1016/j.actao.2014.11.002
Muylaert, R. L., Stevens, R. D., & Ribeiro, M. C. (2016). Threshold effect of habitat loss on bat richness in cerrado-forest landscapes. Ecological Applications, 26, 1854–1867. https://doi.org/10.1890/15-1757.1
O’Farrell, M. J., & Gannon, W. L., (1999). A comparison of acoustic versus capture techniques for the inventory of bats. Journal of Mammalogy, 80, 24–30. https://doi.org/10.2307/1383204
O’Shea, T. J., Cryan, P. M., Hayman, D. T., Plowright, R. K., & Streicker, D. G. (2016). Multiple mortality events in bats: a global review. Mammal Review, 46, 175–190. https://doi.org/10.1111/mam.12064
Park, K. J. (2015). Mitigating the impacts of agriculture on biodiversity: bats and their potential role as bioindicators. Mammalian Biology, 80, 191–204. https://doi.org/10.1016/j.mambio.2014.10.004
Pinto, N., & Keitt, T. H. (2008). Scale-dependent responses to forest cover displayed by frugivore bats. Oikos, 117, 1725–1731. https://doi.org/10.1111/j.1600-0706.2008.16495.x
Reis, N. R., Peracchi, A. L., Lima, I. P., & Pedro, W. A. (2007). Morcegos do Brasil. Londrina: Editora da Universidade Estadual de Londrina.
Ripperger, S. P., Kalko, E. K., Rodríguez-Herrera, B., Mayer, F., & Tschapka, M. (2015). Frugivorous bats maintain functional habitat connectivity in agricultural landscapes but rely strongly on natural forest fragments. Plos One, 10, e0120535. https://doi.org/10.1371/journal.pone.0120535
Sikes, R. S., Gannon, W. L., & the Animal Care and Use Committee of the American Society of Mammalogists. (2016). Guidelines of the American Society of Mammalogists for the use of wild mammals in research. Journal of Mammalogy, 92, 235−253. https://doi.org/10.1644/10-mamm-f-355.1
Silva, C. R., & Bernard, E. (2017). Bioacoustics as an important complementary tool in bat inventories in the Caatinga drylands of Brazil. Acta Chiropterologica, 19, 409–418. https://doi.org/10.3161/15081109acc2017.19.2.017
Smith, H. F., & Sullivan, C. A. (2014). Ecosystem services within agricultural landscapes—Farmers’ perceptions. Ecological Economics, 98, 72–80. https://doi.org/10.1016/j.ecolecon.2013.12.008
Suárez-Rubio, M., Ille, C., & Bruckner, A. (2018). Insectivorous bats respond to vegetation complexity in urban green spaces. Ecology and Evolution, 8, 3240–3253. https://doi.org/10.1002/ece3.3897
Symonds, M. R., & Moussalli, A. (2011). A brief guide to model selection, multimodel inference and model averaging in behavioural ecology using Akaike’s information criterion. Behavioral Ecology and Sociobiology, 65, 13–21.
Trevelin, L. C., Silveira, M., Port-Carvalho, M., Homem, D. H., & Cruz-Neto, A. P. (2013). Use of space by frugivorous bats (Chiroptera: Phyllostomidae) in a restored Atlantic forest fragment in Brazil. Forest Ecology and Management, 291, 136–143. https://doi.org/10.1016/j.foreco.2012.11.013
Voigt, C. C., & Kingston, T., (2016). Bats in the Anthropocene: conservation of bats in a changing world. Cham: Springer International Publishing. https://doi.org/10.1007/978-3-319-25220-9
Weyland-Vieira, M. C., Carauta, J. P. P., Novaes, A., Ataide, E. M., & Martins, E. S. (2014). Vegetação, flora e a conservação das espécies na Fazenda Lagoa. In R. S. L. Laurindo, R. L. M. Novaes, & M. C. Weyland-Vieira (Eds.), RPPN Fazenda Lagoa: educação, pesquisa e conservação da natureza (pp. 75–110). Monte Belo: Instituto Sul Mineiro de Estudos e Conservação da Natureza.
Williams-Guillén, K., & Perfecto, I. (2010). Effects of agricultural intensification on the assemblage of leaf-nosed bats (Phyllostomidae) in a coffee landscape in Chiapas, Mexico. Biotropica, 42, 605–613. https://doi.org/10.1111/j.1744-7429.2010.00626.x
Wickramasinghe, L. P., Harris, S., Jones, G., & Vaughan-Jennings, N. (2004). Abundance and species richness of nocturnal insects on organic and conventional farms: effects of agricultural intensification on bat foraging. Conservation Biology, 18, 1283–1292. https://doi.org/10.1111/j.1523-1739.2004.00152.x