Ectomycorrhizal inoculation with edible fungi increases plant growth and nutrient contents of Pinus ayacahuite
Cristina Arteaga-León a, Jesús Pérez-Moreno a, *, David Espinosa-Victoria a, Juan José Almaraz-Suárez a, Hilda Silva-Rojas a, Adriana Delgado- Alvarado b
a Colegio de Postgraduados, Km 36.5 carretera México-Texcoco, Campus Montecillo, 56230 Texcoco, Estado de México, Mexico
b Colegio de Postgraduados, Campus Puebla, Km 125.5 carretera Federal México-Puebla, 72760, Puebla, Puebla, Mexico
*Corresponding author: jperezm@colpos.mx (J. Pérez-Moreno)
Abstract
The international trade of natural Christmas trees generates billions of US dollars annually; the production of such trees in Mexico is a growing industry, delivering around 900,000 trees annually, mainly from the native species Pinus ayacahuite. To survive, this species establishes a mutualistic symbiosis in its roots known as ectomycorrhiza. However, currently no studies have been performed regarding the ectomycorrhizal inoculation effects on P. ayacahuite. In this work, the effect of ectomycorrhizal inoculation on the growth and nutritional content of P. ayacahuite was evaluated using 2 native edible ectomycorrhizal mushrooms. After 2 years, the inoculation produced increases of total dry weight and total contents of macro- (N, P and Mg) and micronutrients (Fe) of P. ayacahuite. The mycorrhization percentage of inoculated plants varied between 41% and 59%, depending on the inoculum source. Ectomycorrhizal colonization was confirmed with optical and electron microscopy. To our knowledge, this is the first report on the effect on growth and nutrient mobilization by ectomycorrhizae in P. ayacahuite; and one of the first reports on Fe mobilization in gymnosperms by ectomycorrhizal fungi. Our work also demonstrates the importance of inoculation with Helvella cf. lacunosa and Hebeloma mesophaeum for the production of P. ayacahuite plants in nurseries.
Keywords:
Pinaceae; Edible ectomycorrhizal mushrooms; Mycorrhizal fungi; Iron; Natural Christmas trees; Forest biotechnology
© 2018 Universidad Nacional Autónoma de México, Instituto de Biología. Este es un artículo Open Access bajo la licencia CC BY-NC-ND
La inoculación ectomicorrízica con hongos comestibles incrementa el crecimiento vegetal y el contenido nutrimental de Pinus ayacahuite
Resumen
El comercio internacional de árboles de Navidad naturales genera miles de millones de dólares anualmente y la producción de estos árboles en México es una industria en crecimiento que produce alrededor de 900,000 árboles al año, principalmente de la especie nativa Pinus ayacahuite. Para sobrevivir, esta especie establece una simbiosis mutualista en sus raíces conocidas como ectomicorriza. Sin embargo, actualmente no se han realizado estudios con respecto a los efectos de la inoculación ectomicorrízica en P. ayacahuite. En este trabajo se evaluó el efecto de la inoculación ectomicorrízica en el crecimiento y el contenido nutricional de P. ayacahuite utilizando 2 hongos nativos ectomicorrízicos comestibles. Después de 2 años, la inoculación produjo aumentos del peso seco total y del contenido total de macro (N, P y Mg) y micronutrientes (Fe) en P. ayacahuite. El porcentaje de micorrización de las plantas inoculadas varió entre 41% y 59%, dependiendo de la fuente de inóculo. La colonización ectomicorrízica fue confirmada con microscopía óptica y electrónica. Hasta donde conocemos, este es el primer reporte relacionado con el efecto en el crecimiento y la movilización de nutrientes por ectomicorrizas en P. ayacahuite, y uno de los pocos registros de la movilización de Fe en gimnospermas por hongos ectomicorrízicos. El trabajo también demuestra la importancia de la inoculación con Helvella cf. lacunosa y Hebeloma mesophaeum para la producción de plantas de P. ayacahuite en viveros.
© 2018 Universidad Nacional Autónoma de México, Instituto de Biología. This is an open access article under the CC BY-NC-ND license
Palabras clave:
Pinaceae; Hongos ectomicorrízicos comestibles; Hongos micorrícicos; Hierro; Árboles de Navidad naturales; Biotecnología forestal
Introduction
Christmas tree production on a global scale is an industrial activity that generates billions of dollars annually. The demand in Europe and the US for these trees generates 50 and 24.5 million USD per year, respectively (FAO, 2013). This demand favors the reactivation of local economies involved in Christmas tree production primarily by fostering job growth; in the United States of America alone, the industry generates approximately 100,000 jobs annually, but also by contributing to the capture of carbon, replenishment of aquifer mantles, reduction of soil erosion and conservation of biodiversity, especially in degraded areas (NCTA, 2017). Globally, the conifers that are most widely used as Christmas trees belong to Abies, Pinus, and Pseudotsuga genera. To survive, each of these trees requires a mutual association between fungi and tree roots, which is known as ectomycorrhiza. This association increases the absorption of water and organic and inorganic nutrients in the trees because it constitutes a functional extension of their roots, which provides broader soil volume exploration, whereas the associated fungi receive carbon from their host. In a recent study, Bravo et al. (2017) demonstrated that in the case of arbuscular mycorrhiza, lipids are also delivered from the plants to the associated fungi. Moreover, ectomycorrhizal symbiosis provides protection to the plants against pathogens and greater endurance under stress conditions, such as during transplant, drought, high temperature and presence of heavy metals (Read & Pérez-Moreno, 2003). One criterion of ectomycorrhizal fungi selection for forest tree inoculation that has currently gained broad interest at the global scale is the edibility of the fungi (Guerin-Laguette et al., 2014).
Because of their economic, social and environmental relevance, Christmas tree production is an activity of great interest in developing countries that have social, economic and deforestation problems, such as Mexico. Therefore, the development of Christmas tree plantations in Mexico has increased considerably in recent decades; however, the national demand is currently too great to be satisfied by solely the production from domestic plantations. In Mexico, approximately 1.8 million Christmas trees are consumed each year, whereas the annual domestic production ranges between 700,000 and 900,000 trees, requiring national imports, which are mainly from the USA, of nearly 1 million trees each year (Conafor, 2013). In addition to the losses in local and regional customer spending, tree imports may represent a phytosanitary risk to Mexico, which is particularly worrisome because Mexico is an important genetic reservoir at the international level for many forest species. For example, it is known that Mexico hosts the greatest native pine and oak global diversity, with 72 taxa and 150 species of Pinus and Quercus, respectively. Additionally, over 40% of the known fungi genera in the world have been registered in Mexico. The main species used as a Christmas tree in Mexico is the native Pinus ayacahuite (Conafor, 2013). However, despite the large economic, ecological and cultural importance of these trees, there have been no studies on the effect of ectomycorrhizal fungi inoculation on the growth and nutrient contents of P. ayacahuite. Helvella cf. lacunosa and Hebeloma mesophaeum are 2 edible ectomycorrhizal mushrooms naturally associated with P. ayacahuite with great economic, cultural and social importance in central Mexico where they are abundantly marketed (Pérez-Moreno et al., 2010). In addition, despite the fact that macronutrient mobilization via ectomycorrhizal mycelium to their host plants has been widely documented, the transfer of micronutrients by ectomycorrhizae has received in general less attention. Hence, the objective of the present study was to evaluate the influence of ectomycorrhizal inoculation on the growth and macro- (N, P, K, Ca and Mg) and micronutrient (Mn and Fe) content of the Mexican Christmas tree, using Helvella cf. lacunosa and Hebeloma mesophaeum, 2 edible ectomycorrhizal fungi widely consumed and marketed in Central Mexico. For comparison, these inoculation effects were also evaluated when using native soil from a P. ayacahuite orchard as inoculum.
Materials and methods
To obtain seeds, strobili were collected from a Pinus ayacahuite forest stand (Fig. 1a) in Texcoco, Estado de México, Mexico, and were dried at room temperature. Once obtained, the seeds were placed in a water container for 24 hr, with water changes every 7 h to allow for oxygenation. The seeds were then surface sterilized with 30% H2O2 for 20 minutes and washed with sterile distilled water under aseptic conditions. Afterwards, the seeds were sown in growth containers with sterile sand and volcanic rock (known as tezontle). Both the sand and the tezontle were sterilized in advance by steam autoclave at 125 °C for 3 hours.
Concurrently, sporomes were collected from the ectomycorrhizal fungi Helvella cf. lacunosa (Fig. 1b) and Hebeloma mesophaeum from the same forest stand. Stipes were cut to separate them from their pilei because these structures contain the spores. The pilei were dried at 35 °C for 48 hr. Once dry, they were milled and passed through a 1.19-mm sifter. Inocula acquired by this process were stored at 5 °C until use. Simultaneously, material was collected from the fermentation (F) and organic (O) soil horizons from the same P. ayacahuite forest stand where the strobili were collected. This material was used as an ectomycorrhizal inoculum source (hereafter named “native soil”), to homogenize its particle size it was sifted and then stored at 5 °C until use.
Once the Pinus ayacahuite sprouts germinated, they were transferred to 140-cm3 black plastic tubes containing a substrate that was a mix of riverbed sand, ground pine cortex and forest soil in a 2:2:1 proportion. This substrate was sterilized in advance by steam autoclave at 125 °C for 3 hours, laid to rest for 2 days and sterilized once more by steam autoclave for 2 hours on the fourth day. The bottoms of plastic tubes were filled with a layer of sterile tezontle and then topped off with sterile substrate.
Ectomycorrhizal propagule inoculation occurred during sprout transplantation with a 106-108 spore titer per plant of Helvella cf. lacunosa and Hebeloma mesophaeum in 30 cm3 of native soil. Spore dosages from H. cf. lacunosa and H. mesophaeum were applied in 2 steps: the first step occurred during transplantation and the second step occurred 90 days later. To incorporate these inocula, a hole on the substrate next to each plant’s stem was used. For native soil inoculation, the tubes were filled with sterile layers as follows: a base layer of tezontle, then a substrate layer, native soil inoculum, another substrate layer and a final tezontle layer; the approximate layer volumes were 15, 40, 30, 40, and 15 cm3, respectively. In addition, control plants without inoculation were also transplanted in a parallel set in the same substrate than that used for inoculated plants. In summary, there were 4 inoculation treatments: i) H. cf. lacunosa, ii) H. mesophaeum, iii) native soil, and iv) non-inoculated control. A sample size of 15 experimental units (1 plant per unit) was used for each treatment for a total sample of 60 units. To prevent damping-off effects, irrigation included 2.5 g/L Captan every third day until stem lignification; afterwards, the plants were switched to Captan-free irrigation for the next 720 days.
The stem base diameter and height of plants were evaluated in all the samples 720 days after seeding. At that point, the ectomycorrhizal colonization was also evaluated by destructive sampling of 5 randomly selected plants per group, and the aerial zones were separated from root zones. Roots were split into 3 segments corresponding to the superior, central and inferior regions of the root ball, and each of them occupied approximately 47 cm³ of substrate. These roots were washed under running water through 1.19, 0.180 and 0.050 mm sifters to collect most of the short root segments. Counts of mycorrhized, non-mycorrhized and dead roots were performed using a Motic SMX-143 stereoscopic microscope. The colonization percentage was calculated as the ratio of ectomycorrhizal to the total number of observed fine roots from each plant. Transversal sections were screened to determine the presence of ectomycorrhizal roots by morphotyping and through visual confirmation of the mantle, Hartig net and external mycelium. Photographs of these structures were acquired with an Olympus BX51 microscope. Scanning electron microscopy (SEM) analysis of ectomycorrhizal roots for all treatments was performed using a JEOL JSM-6360LV scanning electron microscope (Tokyo, Japan). The dry weight of both the aerial and root zones were evaluated on 10 random samples per group, different than those 5 used to evaluate mycorrhization. Both zones were separately dehydrated at 70 °C for 48 hr. Later, nutritional assays were performed on each zone to screen for macronutrient and micronutrient content (N, P, K, Ca, Mg, Mn and Fe). Nitrogen content was screened by the semimicro-Kjeldahl method (Bremmer, 1975); total phosphate was screened by the method by Allen et al. (1997); potassium was screened by ammonium acetate extraction and flame photometry, and Ca, Mg, Fe, and Mn were screened by atomic absorption spectrophotometry (Varian, Spectra-AA220) in the Soil Fertility Laboratory of Colegio de Postgraduados in Texcoco, Estado de México.
Data analyses for growth, dry weight and nutritional content from both the aerial and root zones were performed using a random experimental design. For all variables, an analysis of variance (Anova) and post hoc Tukey’s pairwise mean comparisons were performed using the Statistical Analysis System software package (SAS, 2002). Root-square transformations of the ectomycorrhizal colonization percentage were included for sample normality.
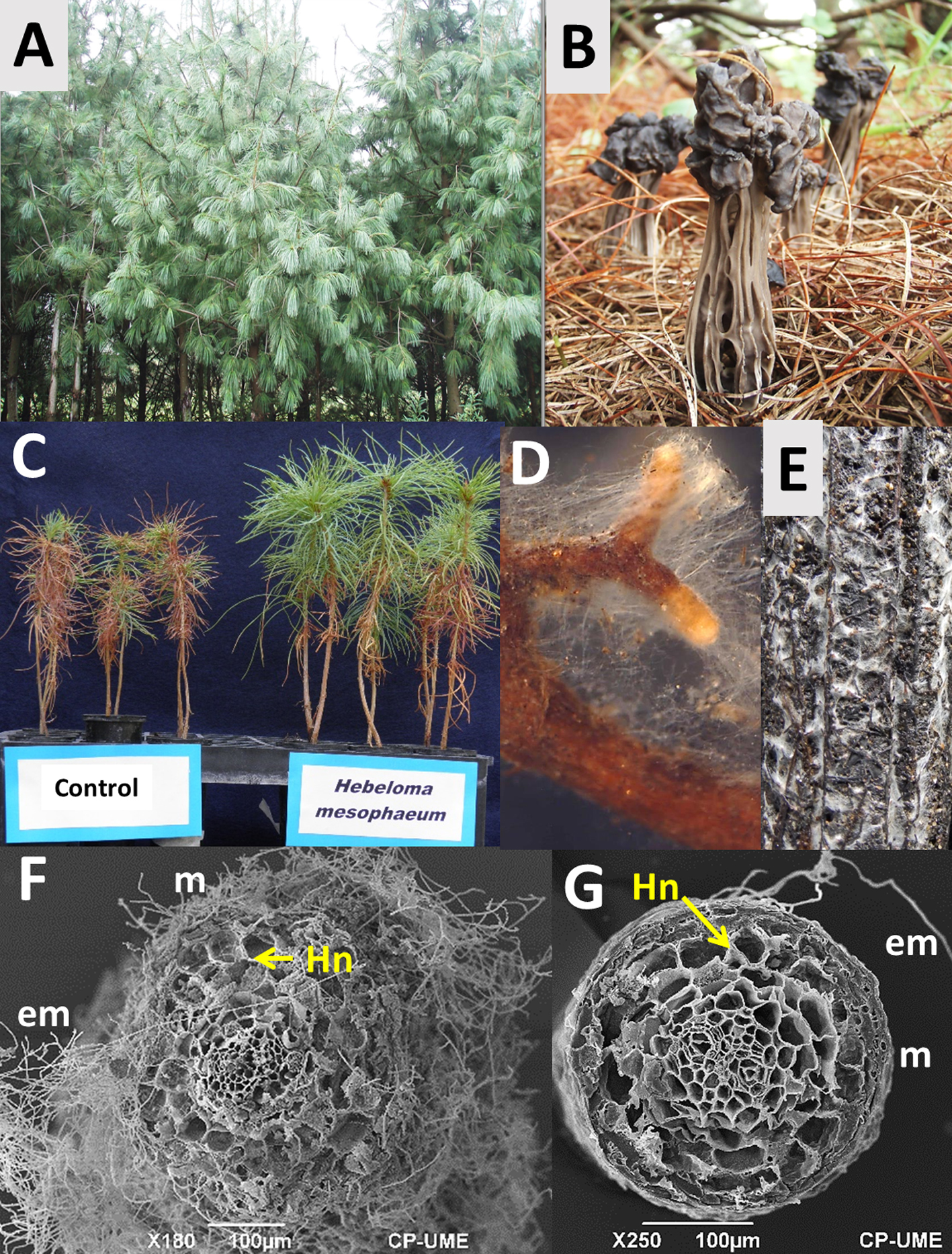
Results
Plant growth. The highest aerial and total dry weight was observed on Helvella cf. lacunosa inoculated plants with respect to native soil and control groups (Tukey p ≤ 0.05) (Table 1). A similar trend was observed only for the total dry weight for H. mesophaeum versus non-inoculated plants (Tukey p ≤ 0.05) (Fig. 1c). In contrast, there were no differences in the root dry weight among inoculated and control groups. The plant heights for all the inoculation conditions exceeded those of the control group (Tukey p ≤ 0.05). An opposite trend was observed in stem diameter without significant differences between treatments (Table 1).
Macronutrients. The aerial parts displayed higher N, P, Ca and Mg contents in all the independent inoculation groups with respect to the control (Tukey p ≤ 0.05) (Table 2). However, the root zones were not significantly distinct among any of the experimental groups. In the case of K, neither the aerial nor root zones were different among the treatments. In contrast, the Ca content distinctions between the inoculated and control plants were particularly interesting. Fold differences in the Ca content of 3.30, 2.64 and 2.37 were found in the plant shoots inoculated with Helvella cf. lacunosa, native soil and Hebeloma mesophaeum, respectively, in comparison with their non-inoculated counterparts (Table 2); higher N contents were also shown in the inoculated plants (Tukey p ≤ 0.05). In contrast, an opposite and non-significant trend was observed for the total K and Ca among the treatments. Helvella cf. lacunosa inocula yielded higher total contents against the non-inoculated plants in both P and Mg, whereas plants inoculated with native soil yielded higher total contents of P (Table 2).
Micronutrients. In the case of Fe content, the trends resembled those in Ca. Moreover, the Fe contents in aerial zones of all of the inoculated groups exceeded those in the control group (Tukey p ≤ 0.05) (Table 3). Nonetheless, differences in the root and total Fe contents were absent among the groups. Differences in the aerial Fe of 3.79, 2.36 and 2.24 from Helvella cf. lacunosa, native soil and Hebeloma mesophaeum groups were detected against the controls (Table 3). In the case of Mn, there were no differences among groups regardless of the inoculum source (Table 3).
Aerial:root zone ratio. The highest dry weight aerial: root zone ratios occurred in plants inoculated with H. cf. lacunosa or native soil with respect to either H. mesophaeum or non-inoculated plants (Table 1). The maximum relative ratio between the groups was found in H. cf. lacunosa versus control comparisons (Table 1). The aerial:root N, Mg and Mn ratios were all higher across all of the inocula. The relative ratio maxima between the inoculated and control groups were largest in the case of Ca and Fe regardless of inoculum source (Tables 2, 3). These observations were particularly evident in H. cf. lacunosa versus non-inoculated plants, with values of 2.69 and 2.87-fold for Ca and Fe, respectively. Similar measurements were observed in the native soil versus control cases, with a relative ratio with a 2.07-fold difference in the aerial:root relative content ratio.
In the present work the aerial:root Fe and Ca content ratios were 1.87-2.87 times greater in the inoculated plants compared to the non-inoculated plants. This range was dependent on the type of inoculum used, which is an indicator of the high efficiency of the employed ectomycorrhizal inocula (H. cf. lacunosa, H. mesophaeum and native soil), not only for soil Fe and Ca uptake but also for mobilization to aerial parts of the inoculated hosts. In the specific case of Fe, the greatest transfer efficiency was observed with H. cf. lacunosa and H. mesophaeum inocula, which was shown by the 2.82- and 2.30- fold gains, respectively, in the aerial:root relative ratios in the inoculated versus non-inoculated plans.
Table 1
Dry weight, height and stem diameter of 2-year-old Pinus ayacahuite plants, inoculated or not, with 3 sources of inoculum.
Treatment |
Dry weight (mg) |
Height (cm) |
Stem |
|||
Shoot |
Root |
Total |
diameter (mm) |
|||
Plants inoculated with Helvella cf. lacunosa |
2360.0±292a |
1536.7±119a |
3896.7±228a |
21.37±0.1a |
3.62±0.1a |
|
Hebeloma mesophaeum |
1692.6±90ab |
1653.1±197a |
3345.7±274a |
18.70±0.4a |
3.71±0.2a |
|
native soil |
1472.6±43b |
1185.8±105a |
2658.4±101ab |
21.47±0.1a |
3.11±0.1a |
|
Non-inoculated plants |
1058.7±40b |
993.4±398a |
2052.1±412.3b |
15.62± 0.4b |
3.79 ±0.2a |
Data are means ± standard error of the mean. n = 10 for dry weight, and n = 15 for height and stem diameter. Values in the same column with the same letter are not different according to the Tukey test (p ≤ 0.05).
Table 2
Shoot, root and total contents of the macronutrients N, P, K, Ca and Mg of 2- year-old Pinus ayacahuite plants, inoculated or not, with 3 sources of inoculum.
Treatment |
N |
P |
K |
Ca |
Mg |
(mg per plant) |
|||||
Shoot of plants inoculated with Helvella cf. lacunosa |
12.98±1.60a |
0.70±0.087a |
5.90±0.73a |
10.85±1.34a |
5.04±0.78a |
Hebeloma mesophaeum |
13.37±0.71a |
0.67±0.03a |
4.40±0.23a |
7.78 ±0.41a |
4.06±0.21a |
native soil |
11.33±0.32a |
0.88±0.25a |
6.33±0.18a |
8.67±2.73ba |
3.23±0.09ba |
non-inoculated plants |
5.82±0.21b |
0.42±0.01b |
4.22±1.02a |
3.28±0.12b |
2.01±0.07b |
Root of plants inoculated with Helvella cf. lacunosa |
11.37±0.87a |
0.92±0.07a |
3.22±0.24a |
11.21±0.86a |
3.68±0.28a |
Hebeloma mesophaeum |
10.91±1.30a |
0.82±0.98a |
4.13±0.49a |
11.57±1.38a |
2.81±0.33a |
native soil |
6.04±0.39a |
0.94±0.08a |
2.37±0.21a |
11.02±0.97a |
2.60±0.23a |
non-inoculated plants |
6.45±2.58a |
0.49±0.19a |
2.18±0.87a |
9.14±3.66a |
2.28±0.91a |
Total contents of plants inoculated with Helvella cf. lacunosa |
24.35±1.18a |
1.62±0.06a |
9.12±0.58a |
22.07±0.97a |
8.73±0.62a |
Hebeloma mesophaeum |
24.28±1.91a |
1.50±0.12ab |
8.53±0.69a |
19.35±1.72a |
6.87±0.52ba |
native soil |
17.38±0.22a |
1.83±0.08a |
8.70±0.23a |
19.69±1.83a |
5.84±0.22b |
non-inoculated plants |
12.28±2.66b |
0.92±0.20b |
6.40±1.27a |
12.42±3.70a |
4.29±0.94b |
Data are means ± standard error of the mean. n = 3. Values in the same column with the same letter, for each part of the plant, are not different according to the Tukey test (p ≤ 0.05).
Table 3
Shoot, root and total contents of the micronutrients Fe and Mn of 2-year-old Pinus ayacahuite plants, inoculated or not, with 3 sources of inoculum.
Treatment |
Shoot |
Root |
Total |
Fe (μg) |
|||
Plants inoculated with Helvella cf. lacunosa |
1.86±0.23a |
35.62±2.75a |
37.48±2.60a |
Hebeloma mesophaeum |
1.11±0.05b |
26.61±3.17a |
27.73±3.22a |
native soil |
1.16±0.03b |
32.37±2.86a |
33.53±2.85a |
non-inoculated plants |
0.49±0.01c |
27.27±10.93a |
27.77±10.93a |
Mn (μg) |
|||
Plants inoculated with Helvella cf. lacunosa |
6.03±1.33a |
2.25±0.17a |
8.29±1.20a |
Hebeloma mesophaeum |
5.28±0.28b |
1.86±0.22a |
7.14±0.47a |
native soil |
1.92±0.80b |
0.90±0.08a |
2.83±0.86b |
non-inoculated plants |
5.24±0.19ab |
1.33±0.53a |
6.57±0.62ab |
Data are means± standard error of the mean. n = 3. Values in the same column with the same letter are not different according to the Tukey test (p ≤ 0.05).
Total fine root counts were higher in the H. cf. lacunosa group with respect to the non-inoculated group (Fig. 2). The total root numbers were 3.65-fold higher in this treatment group versus control. In contrast, neither H. mesophaeum nor the native soil inoculated plants displayed differences compared with control conditions. The dead fine root percentages ranged by approximately 4.0, 16.1, 26.3 and 31.3% for plants inoculated with H. cf. lacunosa, H. mesophaeum and native soil and non-inoculated plants, respectively. Morphotyping showed that H. cf. lacunosa resulted in 2.2- and 3.5-times higher mycorrhized roots than H. mesophaeum and native soil, respectively (Fig. 2). The mycorrhizal colonization in H. cf. lacunosa, H. mesophaeum and native soil groups was 58.7, 52.3 and 40.9%, respectively after 2 years of growth in greenhouse conditions, whereas the non-inoculated plants displayed a low percentage of ectomycorrhizal colonization, mostly by Cenococcum cf. geophilum, according to morphotyping (4%). In the work presented here, ectomycorrhizal colonization for P. ayacahuite with the H. cf. lacunosa ascomycete and H. mesophaeum basidiomycete is presented for the first time (Fig. 1d, e, f, g).
Pinus ayacahuite with Hebeloma mesophaeum: ectomycorrhiza with orange-brown to red brown surface in the base and light brownish to cream toward the apex, simple and more frequently dichotomous, from 0.2 to 1.0 mm in diameter and up to 13 mm in length, with a plectenchymaotus poor developed mantle densely cottony, and a well-developed Hartig net entering the interstitial spaces of the first 2 or 3 layers of epidermal cells, with abundant emanating hyphae, with frequent clamp-connections, ramified, lax, visible macroscopically, rhizomorphs absent (Fig. 1D, E, F). Similar but smaller mycorrhizas were reported by Carrasco-Hernández et al. (2015).
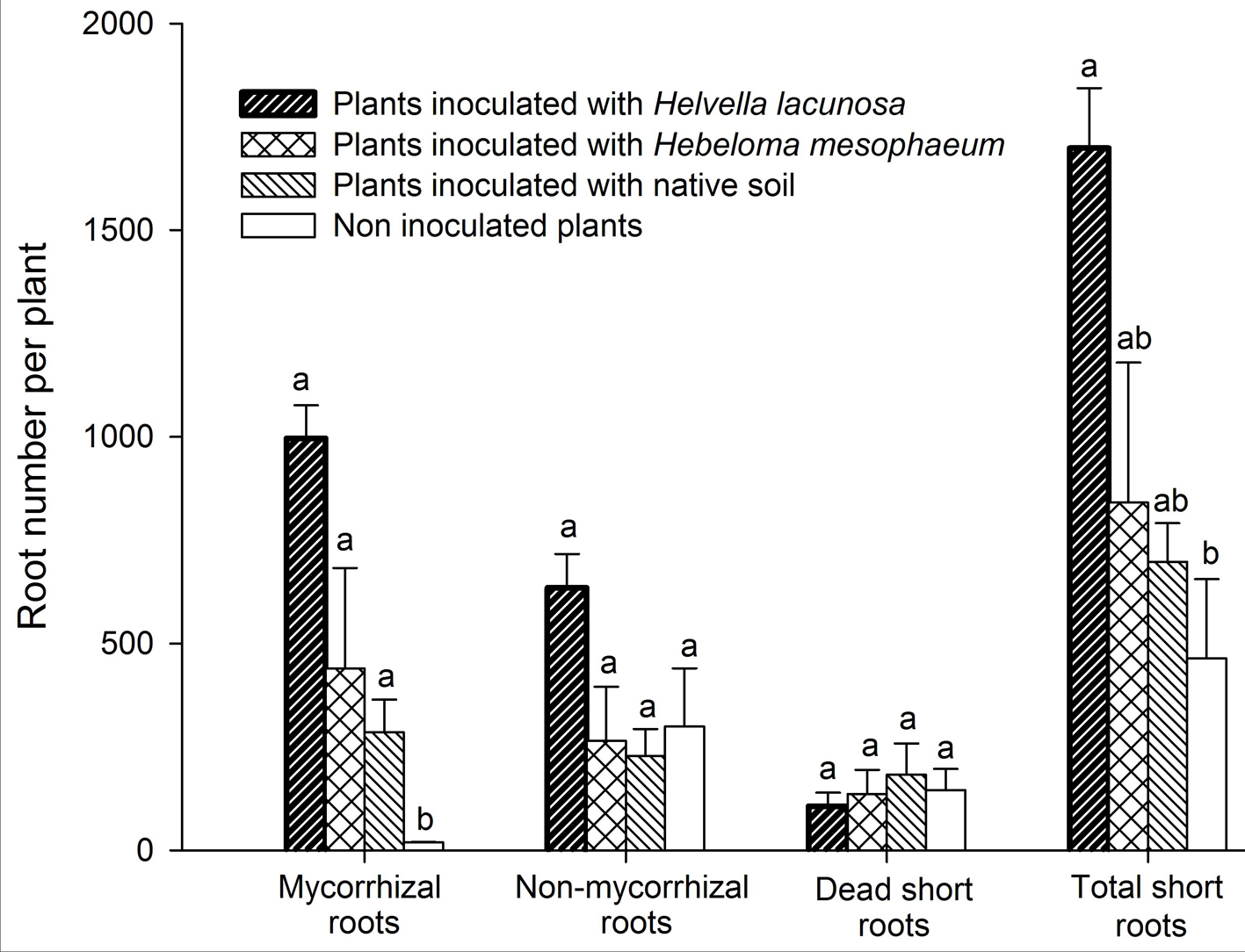
Pinus ayacahuite with Helvella cf. lacunosa: ectomycorrhizae dark brown in the base, becoming lighter toward the apex with orange-brown to red brown surface, whitish to cream tips in young stages, smooth, simple or dichotomous, from 0.3 to 0.7 mm in diameter and up to 10 mm in length, with a pseudoparenchymatous mantle and a well-developed. Hartig net entering the interstitial spaces of the first 2 or 3 layers of epidermal cells, clamps were not observed, and there was scarce, thick, stout, thick walled external hyphae and the rhizomorphs were absent (Fig. 1G). A similar morphoanatomical structure was described for Helvella spp. by Tedersoo et al. (2006).
Discussion
Previously, it had been documented that ectomycorrhizal fungi inoculation led to positive effects on the growth and nutritional content of their associated host plants, which were both gymnosperms and angiosperms (Siddiqui & Pitchtel, 2008). Recently, edibility has been included as a selection criterion for ectomycorrhizal fungi used for plant inoculation related to forest species (Guerin-Laguette et al., 2014) because in addition to the beneficial effects from edible ectomycorrhizal fungi inoculation, the international trade of these mushrooms has been valued in billions of dollars per year (Hall et al., 2003). It has also been demonstrated that edible ectomycorrhizal fungi constitute a non-timber forest product of enormous social, environmental and economic importance to forest ecosystem conservation. Sousa et al. (2012) inoculated Pinus pinaster plants with the edible ectomycorrhizal fungi Suillus bovinus, Laccaria laccata and Lactarius deterrimus and reported favorable effects in terms of plant height, total biomass and greater N concentration in the aerial parts of inoculated plants compared with the non-inoculated plants. Slama et al. (2012) inoculated Helianthemum sessiliflorum plants, a shrub species from African desert regions, with the edible ectomycorrhizal fungus Terfezia boudieri, which is also known as the “desert truffle”. These authors found that inoculated plants presented better development in terms of height, leaf counts and N, P and K concentrations compared with non-inoculated plants. Sánchez-Zabala et al. (2013) inoculated Pinus pinaster with edible Suillus luteus and Lactarius deliciosus ectomycorrhizal fungi and reported increased plant height and stem diameter for both fungi compared to non-inoculated plants. Three years after a nursery-to-field transplantation, these authors observed that the inoculated plants displayed lower mortality indices with respect to non-inoculated indices. In addition, they found that levels of soluble carbohydrates and amino acids were higher during cold climate seasons in inoculated plants, which contributed to overcoming potential freeze shock-associated damage in vegetal tissues. For those reasons, these authors indicated that the greater nutritional content found in inoculated plants conferred a competitive advantage and allowed them to prevail over transplantation-related stress upon relocation from the nursery to fields compared to non-inoculated plants. In the case of Mexico, currently there is no information related with the influence of controlled ectomycorrhization of any native tree species under field conditions on nutrient contents or mobilization, despite the fact that this is an urgent need to understand the physiological changes induced by ectomycorrhizal symbiosis in order to increase field survival. The only exception is the report of survival increases in Central Mexico induced in plants of Pinus pringlei inoculated with Hebeloma alpinum, Laccaria trichodermophora and Thelephora terrestris, compared with non-inoculated plants, recently published (López-Gutiérrez et al., 2018).
Fe is a fundamental micronutrient for basic cellular processes such as photosynthesis, respiration and DNA synthesis. Despite its abundance in the earth’s cortex, Fe availability in aerobic environments is frequently limited because of its insoluble presentation (Haselwandter et al., 2011). It has been shown that ectomycorrhizal fungi can produce low molecular weight compounds (< 1,500 Da) with a high affinity for ionic Fe, which are known as siderophores (Landerweert et al., 2001; Szaniszlo et al., 1981). The types of siderophores produced by ectomycorrhizal fungi belong to the hydroxamate group. Szaniszlo et al. (1981) demonstrated that diverse ectomycorrhizal fungi in the Amanita, Boletus, Suillus, Pisolithus, and Cenoccocum genera release hydroxamate siderophores under limiting Fe conditions. Haselwandter and Winkelmann (2002) found that the Cenoccocum geophilum siderophore belongs to the ferricrocins group; this compound, along with ferrichrome, another siderophore, has also been detected in Suillus variegatus. Similarly, other siderophore groups have been reported in Suillus species, such as S. granulatus, which releases fusigen, ferrichrome, coprogen and triacetyl fusarin C, and S. luteus, which produces fusigen, ferricrocin and coprogen (Haselwandter et al., 2011). Most of these studies have been performed in pure in vitro cultures of ectomycorrhizal fungi, although there have been 2 notable exceptions: works by Rineau et al. (2008) and van Hesse et al. (2006). Rineau and collaborators evaluated roots ectomycorrhized with Xerocomus sp. and Lactarius subdulcis that were freshly collected from mature ecosystems in France to determine their capacity to chelate Fe complexes and characterize free ionic Fe mobilization. In more realistic settings than in vitro settings, these authors found that Xerocomus ectomycorrhizae were more efficient in accessing Fe complexes and free ions than those found with L. subdulcis. In the case of the Hebeloma genus, which was evaluated here, its siderophore production was previously reported by van Hees et al. (2006). These authors demonstrated that the external mycelium of H. crustuliniforme grown symbiotically with Pinus sylvestris can produce ferricrocin as one of its exuded compounds. Although all known organisms require Fe to perform a multitude of metabolic processes, the overabundance or excess storage of Fe can be toxic, so it requires precisely regulated acquisition and storage biological systems. Ferricrocin has been shown to be involved in intra- and extra-cellular Fe distribution, so Ferricrocin intervenes in Fe regulation. In general terms, Fe mobilization by ectomycorrhizal fungi in gymnosperms has received scant attention. To our knowledge, the presented work is one of the first showing mobilization of this micronutrient into gymnosperms inoculated with ectomycorrhizal fungi.
As far as we know the present work registers the ectomycorrhizal colonization of P. ayacahuite with the H. cf. lacunosa ascomycete and H. mesophaeum basidiomycete for the first time. The Helvella genus includes 52 species (Kirk et al., 2008), 19 of which have been reported in Mexico. One such species is H. lacunosa, which has been reported in Europe, North America and Asia. This species is highly esteemed as an edible fungus in Mexico and is abundantly marketed in the central regions of the country (Pérez-Moreno et al., 2008; 2010) where it is found in association with Pinus, Abies, and mixed Quercus forests. Zhang et al. (2010) isolated an alkaline protease termed “helvellisin” from H. lacunosa sporophores. Alkaline proteases are widely used commercial enzymes in the detergent, brewery, photographic and dermatological industries as well as in protein, meat and dairy good production. Recently, Leal et al. (2013) reported a high lycopene concentration in this ectomycorrhizal fungus. Lycopenes are compounds with antioxidant, anti-inflammatory and chemotherapeutic properties against cardiovascular disease, neurodegenerative illness and certain types of cancer, and they have also been used to treat male infertility. Despite the relevance of H. lacunosa, to our knowledge, there is only 1 published study that has evaluated the effects of its inoculation in trees. Martínez-Amores et al. (1990) reported an increase in the aerial zone dry weight in Pinus patula 12 months post-inoculation. In the work presented here, the edible ectomycorrhizal fungus H. lacunosa was used to inoculate P. ayacahuite and produced highly favorable results with respect to the increased aerial and total dry weights as well as the higher P, Mg and Fe concentrations and greater fine root sprouting compared to non-inoculated plants. The other fungi species used in this research that presented beneficial outcomes in P. ayacahuite was H. mesophaeum. Species from Hebeloma form ectomycorrhizal associations with both angiosperms and gymnosperms in early development in disrupted habitats and mature forests (Nara et al., 2003). Garibay-Orijel et al. (2013) reported that Hebeloma species possess a significant ecological importance in Pinus montezumae ecosystems because the genus was best manifested in spore banks from existing ectomycorrhizal fungi communities in the Trans-Mexican Volcanic Belt. Obase et al. (2007) reported that H. mesophaeum was an important fungal species involved in the settlement of Pinus maximowiczii, Salix hultenii var. angustifolia and Salix sachalinensis following devastation from a local volcanic eruption in Japan. Hebeloma mesophaeum is an ectomycorrhizal fungus with ample commercialization in Central Mexico markets (Pérez-Moreno et al., 2010). Previous work has shown the efficacy of this species in augmenting vegetal growth and nutrient content in Pinus hartwegii (Perea-Estrada et al., 2009), P. gregii, P. patula, and P. pseudostrobus (Carrasco- Hernández et al., 2011; Martínez-Reyes et al., 2012). However, such effects had not been reported in P. ayacahuite a native forest species from Mesoamerica that is valued because it is used as a source of timber, in the production of pulp and paper and artisan crafts and as pellets and shavings. Currently, this species shares a high ecological and economic value in Mexico for commercial Christmas tree plantations and ornamental purposes in gardening and urban spaces (Conafor, 2013). Nonetheless, morphological descriptions of the ectomycorrhizal symbionts linked to P. ayacahuite have received low attention. To date, available reports have only been produced for the synthesis and description of ectomycorrhiza between a pair of Lactarius indigo strains with Pinus ayacahuite (Flores et al., 2005).
Inoculation with the edible ectomycorrhizal fungi H. cf. lacunosa and H. mesophaeum resulted in higher total biomass content in P. ayacahuite. Greater concentrations of N, P, Ca, Mg and Fe occurred in the shoots of the inoculated plants compared with the non-inoculated plants, and this result was independent of the inoculum source. In the case of Fe and Ca, their mobility to plant shoots was aided by ectomycorrhizal inoculation with all our inocula sources. Ectomycorrhizal colonization varied between 41 and 59% depending on the type of inocula, thus showing the efficacy of the implemented methodology. The total fine root production in inoculated Pinus ayacahuite plants with Helvella cf. lacunosa was 3.5 times greater than in plants without inoculation. To our knowledge, this is the first reported work showing the beneficial nutritional effect of ectomycorrhizal inoculation in P. ayacahuite. Additionally, this work is one of the few reports of an increased Fe content in the plant shoots of gymnosperms as a consequence of ectomycorrhizal inoculation. These data demonstrate the potential for the biotechnological use of edible H. cf. lacunosa and H. mesophaeum ectomycorrhizal fungi on the production of the greenhouse-grown Mexican Christmas tree Pinus ayacahuite. Due to its edibility both species of mushrooms studied in the present work are commercialized in dozens of towns in central Mexico, which constitute an important factor in the feasibility to produce some thousands of ectomycorrhizal P. ayacahuite plants with the tested biotechnology. However, it is important to stress that with a massive inoculation of a great number of plants complementary technologies of ectomycorrhizal inoculum production would be necessary, including for example mycelial slurries, which is also an urgent research topic necessary to be developed.
Acknowledgments
The first author thanks the Mexican Council of Science and Technology (Conacyt) for a Ph.D. scholarship. Financial support from the Project Conacyt 246674 is also acknowledged. We thank Magdalena Martínez-Reyes from Colegio de Postgraduados for her valuable suggestions and technical advice. The valuable comments of two anonymous referees and the associated editor are also deeply acknowledged.
References
Allen, S. E., Grimshaw, H. M., Parkinson, J. A., & Quarmbym, C. (1997). Chemical analysis of ecological materials. Oxford: Blackwell Scientific Publications.
Bremmer, J. M. (1975). Total Nitrogen. In C.A. Black (Ed.), Methods of soil analysis. Agronomy Part 2 (pp. 1149-1178). Madison, Wisconsin: American Society of Agronomy.
Bravo, A., Brands, M., Wewer, V., Dörman, P., & Harrison, M. J. (2017). Arbuscular mycorrhiza-specific enzymes FatM and RAM2 fine-tune lipid biosynthesis to promote development of arbuscular mycorrhiza. The New Phytologist, 214, 1631–1645.
Carrasco-Hernández, V., Pérez-Moreno, J., Espinosa, H. V., Almaraz, S. J., Quintero, L. R., & Torres, A. M. (2011). Contenido de nutrientes e inoculación con hongos ectomicorrízicos comestibles en dos pinos neotropicales. Revista Chilena Historia Natural, 11, 83–96.
Carrasco-Hernández, V., Pérez-Moreno, J., Quintero, L. R., Espinosa, S. T., Lorenzana, F. A., & Espinosa, H. V. (2015). Edible species of the fungal genus Hebeloma and two neotropical pines. Pakistan Journal of Botany, 47, 319–326.
Conafor (Comisión Nacional Forestal). (2013). Árboles de Navidad. México City: Secretaría del Medio Ambiente y Recursos Naturales/ Comisión Nacional Forestal.
FAO (United Nations Organization for Food and Agriculture). (2013). Sustainable Christmas trees give German forests a boost. Forestry Department. Infosylva Forestry News Clippings, 24, 15–17.
Flores, R., Días, G., & Honrubia, M. (2005). Mycorrhizal synthesis of Lactarius indigo (Schw.) Fr. with five Neotropical pine species. Mycorrhiza, 15, 563–570.
Garibay-Orijel R., Morales, M. E., Domínguez, G. M., & Flores, G. A. (2013). Caracterización morfológica y genética de las ectomicorrizas formadas entre Pinus montezumae y los hongos presentes en los bancos de esporas en la Faja Volcánica Transmexicana. Revista Mexicana de Biodiversidad, 54, 153–169.
Guerin-Laguette, A., Cummings, N., Butler R. C., Willows, A., Hesom-Williams, N., Li, S., & Wang, Y. (2014). Lactarius deliciosus and Pinus radiata in New Zealand: towards the development of innovative gourmet mushroom orchards. Mycorrhiza, 24, 511–523.
Hall, R. I., Stephenson, L. S., Buchanan, K. P., Yun, W., & Cole, L. A. (2003). Edible and poisonous mushrooms of the world. Christchurch, New Zealand: Institute of Crop & Food Research.
Haselwandter, K., Häninger, G., & Ganzera, M. (2011). Hydroxamate siderophores of the ectomycorrhizal fungi Suillus granulatus and S. luteus. Biometals, 24, 153–157.
Haselwandter, K., & Winkelmann, G. (2002). Ferricrocin: an ectomycorrhizal siderophore of Cenococcum geophilum. Biometals, 15, 73–77.
Kirk, P. M., Cannon, P. F., Minter, D. W., & Spatafora, J. A. (2008). Dictionary of the fungi, 10th Edition. Walingford, UK: CAB International.
Landeweert, R., Hoffland, E., Finlay, R. D., Kuyper, T. W., & Van, B. N. (2001). Linking plants to rocks: ectomycorrhizal fungi mobilize nutrients from minerals. Trends in Ecology and Evolution, 16, 248–253.
Leal, R. A., Barros, L., Barreira, C. M. J., Sousa, J. M., Martins, A., Santos-Buelga, C. et al. (2013). Portuguese wild mushrooms at the “pharma-nutrition” interface: Nutritional characterization and antioxidant properties. Food Research International, 50, 1–9.
López-Gutiérrez, A., Pérez-Moreno, J., Hernández-Santiago, F., Uscanga-Mortera, E., García-Esteva, A., Cetina-Alcalá, V. M. et al. (2018) Nutrient mobilization, growth and field survival of Pinus pringlei inoculated with three ectomycorrhizal mushrooms. Botanical Sciences, 96, 286–304.
Martínez-Amores, E., Valdes, M., & Quintos, M. (1990). Seedling growth and ectomycorrhizal colonization of Pinus patula and P. radiata inoculated with spores of Helvella lacunosa, Russula brevipes or Lycoperdon perlatum. New Forests, 4, 237–245.
Martínez-Reyes, M., Pérez-Moreno, J., Villareal-Ruiz, L., Ferrera-Cerrato, R., Xoconostle-Cázares, B., Vargas-Hernández, J. et al. (2012). Crecimiento y contenido nutrimental de Pinus greggii Engelm. inoculado con el hongo comestible ectomicorrízico Hebeloma mesophaeum (Pers.) Quél. Revista Chapingo Serie Ciencias Forestales y del Ambiente, 18, 183–92.
Nara, K., Nakaya, H., & Hogetsu, T. (2003). Ectomycorrhizal sporocarp succession and production during early primary succession on Mount Fuji. New Phytologist, 158, 193–206.
NCTA (National Christmas Tree Association). (2017). Christmas Tree Statistics. National Christmas Tree Association (NCTA). Washington D.C.: USDA National Agricultural Statistics Science.
Obase, K., Tamai, Y., Yajima, T., & Miyamoto, T. (2007). Mycorrhizal associations in woody plant species at the Mt. Usu vocano, Japan. Mycorrhiza, 17, 209–215.
Perea-Estrada, V. M., Pérez-Moreno, J., Villareal-Ruiz, L., Trinidad-Santos, A., de Bauer, M. L., Cetina-Alcalá, V. M. et al. (2009). Humedad edáfica, nitrógeno y hongos ectomicorrízicos comestibles en el crecimiento de pino. Revista Fitotecnia Mexicana, 32, 93–102.
Pérez-Moreno, J., Lorenzana-Fernández, A., Carrasco-Hernández, V., & Yescas-Pérez, A. (2010). Los hongos comestibles silvestres del parque Nacional Izta-Popo, Zoquiapan y Anexos. Mexico City: Colegio de Postgraduados/ Semarnat/ Conacyt.
Pérez-Moreno, J., Martínez-Reyes, M., Yescas-Pérez, A., Delgado-Alvarado, A., & Xoconostle-Cázares, B. (2008). Wild mushroom markets in Central Mexico and a case study at Ozumba. Economic Botany, 62, 425–436.
Read, J. D., & Pérez-Moreno, J. (2003). Mycorrhizas and nutrient cycling in ecosystems – a journey towards relevance? New Phytologist, 157, 475–492.
Rineau, F., Courty, P. E., Uroz, S., Buée, M., & Garbaye, J. (2008). Simple microplate assay to measure iron mobilization and oxalate secretion by ectomycorrhizal tree roots. Soil Biology and Biochemistry, 40, 2460–2463.
Sánchez-Zabala, J., Majada, J., Martín-Rodrígues, N., González-Murúa, C., Ortega, U., Alonso-Graña, M. et al. (2013). Physiological aspects underlying the improved outplanting performance of Pinus pinaster Ait. seedlings associated with ectomycorrhizal inoculation. Mycorrhiza, 23, 627–640.
SAS (Statistical Analysis System Institute). (2002). SAS User´s guide. Version 9. Cary, North Carolina: SAS Institute.
Siddiqui, Z. A., & Pitchel, J. (2008). Mycorrhizae: an overview. In Z. A. Siddiqui, M. S. Akhtar, & K. Futai (Eds.), Sustainable agriculture and forestry (pp. 1–35). Heidelberg: Springer.
Slama, A., Gorai, M., Fortas, Z., Boudabous, A., & Neffati, M. (2012). Grow, root colonization and nutrient status of Helianthemum sessiliflorum Desf. inoculated with a desert truffle Terfezia boudieri Chatin. Saudi Journal of Biological Science, 19, 25–29.
Sousa, N. R., Franco, A. R., Oliveira, R. S., & Castro, P. M. L. (2012). Ectomycorrhizal fungi as an alternative to the use of chemical fertilisers in nursery production of Pinus pinaster. Journal of Environmental Management, 95 (Suppl.), S269– S274.
Szaniszlo, P. J., Powell, P. E., Reid, C. P. P., & Cline, G. R. (1981). Production of hydroxamate siderophore iron chelators by ectomycorrhizal fungi. Mycologia, 73, 1158–1174.
Tedersoo, L., Hansen, K., Brian, A., Perry, B. A., & Kjøller, R. (2006). Molecular and morphological diversity of Pezizalean ectomycorrhiza. New Phytologist, 170, 581–596.
van Hees, P. A., Rosling, A., Essén, S., Godbold, D. L., Jones, D. L., & Finlay, R. D. (2006). Oxalate and ferricrocin exudation by the extramatrical mycelium of an ectomycorrhizal fungus in symbiosis with Pinus sylvestris. New Phytologist, 169, 367–378.
Zhang, G., Wang, H., Zhang, X., & Ng, T. (2010). Helvellisin, a novel alkaline protease from the wild ascomycete mushroom Helvella lacunosa. Journal of Bioscience and Bioengineering, 109, 20–24.