Arturo Tavera a, Martín H. de Santiago-Hernández a, b, Eduardo Cuevas a, b, *
a Universidad Michoacana de San Nicolás de Hidalgo, Facultad de Biología, Av. Francisco J. Mújica s/n, Ciudad Universitaria, 58030 Morelia, Michoacán, Mexico
b Universidad Nacional Autónoma de México, Unidad Morelia, Escuela de Estudios Superiores, Laboratorio Nacional de Síntesis Ecológica, Antigua Carretera a Pátzcuaro Núm. 8701, Ex Hacienda de San José de La Huerta, 58190 Morelia, Michoacán, Mexico
*Corresponding author: eduardo.cuevas@umich.mx (E. Cuevas)
Received: 16 November 2022; accepted: 17 August 2023
Abstract
The ecological network approach allows the analysis of interactions between plant communities and their floral visitors. However, most floral visitation network studies analyze the interactions with a temporally-static perspective. Hence, few studies have evaluated the temporal variation of these systems. This study characterized the plant-floral visitor interaction network of a temperate forest remnant in Michoacán, analyzing monthly changes in its composition, structure, and dynamics during 3 seasons of the year. In total, we recorded 1,325 floral visits and 131 links between 27 plant species and 74 floral visitor species. Most of the plant species observed belong to the families Lamiaceae, Asteraceae, and Apiaceae, while most of the floral visitor species observed belong to the orders Hymenoptera, Lepidoptera, and Diptera. Most of the floral visits were recorded in highly abundant plant species with longer flowering periods at the study site. The constant species turnover gave rise to monthly plant-floral visitor networks with different levels of specialization, connectance, nestedness, and modularity. Our results suggest that plant-floral visitor networks in temperate forest remnants are highly dynamic and poorly connected, which makes them vulnerable to drastic changes in the environment.
Keywords: Angiosperms; Floral phenology; Rewiring; Species turnover
© 2023 Universidad Nacional Autónoma de México, Instituto de Biología. This is an open access article under the CC BY-NC-ND license
(http://creativecommons.org/licenses/by-nc-nd/4.0/).
Variación temporal de una red planta-visitante floral en un bosque templado de Michoacán, México
Resumen
El enfoque de redes ecológicas permite analizar las interacciones entre comunidades de plantas y sus visitantes florales. Sin embargo, la mayoría de los estudios emplean una perspectiva temporal estática y existen pocos estudios que hayan evaluado la variación temporal de estos sistemas. Este trabajo caracterizó la red planta-visitante floral en un remanente de bosque templado en Michoacán, analizando los cambios mensuales en su composición, estructura y dinámica durante 3 épocas del año. En total, registramos 1,325 visitas florales y 131 vínculos entre 27 especies de plantas y 74 especies de visitantes florales. La mayoría de las plantas observadas pertenecen a las familias Lamiaceae, Asteraceae y Apiaceae, mientras que la mayoría de los visitantes florales observados pertenecen a los órdenes Hymenoptera, Lepidoptera y Diptera. La mayoría de las visitas florales fueron observadas en especies con periodos de floración más largos y mayor abundancia en el sitio de muestreo. El recambio constante de especies dio lugar a redes planta-visitante floral mensuales con diferentes niveles de especialización, conexión, anidamiento y modularidad. Estos resultados sugieren que las redes planta-visitante floral en remanentes de bosque templado son muy dinámicas, poco conectadas y muy vulnerables a cambios drásticos en el ambiente.
Palabras clave: Angiospermas; Fenología floral; Recableado; Recambio de especies
© 2023 Universidad Nacional Autónoma de México, Instituto de Biología. Este es un artículo Open Access bajo la licencia CC BY-NC-ND
Introduction
A high percentage of flowering plants depend on animals for sexual reproduction and floral visitors may facilitate this task by carrying and transferring pollen (Ollerton et al., 2011). The interaction network analysis approach allows for the quantitative description of the interaction between angiosperms and floral visitors at the community level and the relationships they establish, enabling insights into networks’ dynamics and structure (Vizentin-Bugoni et al., 2018). This perspective could be helpful to predict possible community level responses to environmental disturbances (Guzman et al., 2021; Morente-López et al., 2018).
Some studies have described common patterns in most plant-pollinator networks, regardless of their geographic location (Vizentin-Bugoni et al., 2018). It has been suggested that plant-pollinator networks have: 1) a higher proportion of pollinator species than flowering plant species (Olesen & Jordano, 2002); 2) low levels of connectance, i.e., few plant-pollinator links observed out of the total possible (Blüthgen et al., 2008; Jordano et al., 2009); 3) unequal distribution, as not all species interact with the same number of species, and the interactions do not have the same intensity (Soares et al., 2017; Vizentin-Bugoni et al., 2018); 4) a nested structure where specialist pollinators interact with both specialist and generalist plants (Bascompte et al., 2003; Traveset et al., 2016); and 5) a modular structure in which groups of species interact more with each other than with the rest of the species in the network (Dormann & Strauss, 2014; Kaiser-Bunbury & Blüthgen, 2015). Although these are general patterns for plant-pollinator networks, some features are more closely related to geographic factors. Thus, we might expect different scenarios in different places. For example, we can expect a greater number of interacting species in the tropics than in other regions (Gaston, 1996; Willig et al., 2003). In this scenario, connectance, a metric inversely related to network size (Jordano et al., 2009), would have lower values in tropical areas than in non-tropical areas. A higher diversity of pollinator groups is also related to high values of modularity (Vizentin-Bugoni et al., 2018).
In most studies, plant-floral visitor networks are shown from a temporally static perspective where a single moment is analyzed or a whole sampling season is summarized in one interaction matrix. The failure to consider temporal variation in these systems could lead to potential misinterpretations of network parameters, structure, and dynamics, because broad temporal-scale data aggregation implies pooling non co-occurring plant and pollinator species, thereby including forbidden links into network analysis (Jordano, 2016; Jordano et al., 2003; Olesen et al., 2011; Schwarz et al., 2020).
There are few studies exploring the temporal variation of plant-floral visitor networks across different time scales (Alarcón et al., 2008; Baldock et al., 2011; Basilio et al., 2006; Burkle & Irwin 2009; Burkle et al., 2013; Cuartas-Hernández & Medel, 2015; Dupont et al., 2009; Morente-López et al., 2018; Petanidou et al., 2008; Rasmussen et al., 2013; Schwarz et al., 2020; Souza et al., 2018), and most of these studies have been conducted in regions outside the tropics (Souza et al., 2018). Poisot et al. (2012) proposed the partition of networks dissimilarity into 2 main components: species turnover, which refers to the gain or loss of interactions due to phenology-driven changes in the composition of plant and/or pollinator communities, and interaction rewiring, which refers to interactions reassembly between the same plant and pollinator species over time (CaraDonna et al., 2017; Fründ, 2021; Poisot, 2022; Poisot et al., 2012).
The empirical evidence suggests that these β-diversity components affect the structure of plant-pollinator/floral visitor interaction networks over time (Poisot et al., 2012). For example, CaraDonna et al. (2017) evaluated the intra-seasonal change of a plant-pollinator network for 3 years, finding that 20% of the temporal variation was due to species turnover, while 80% was due to interactions rewiring. The scarce evidence in the scientific literature about the temporal variation of plant-pollinator and plant-floral visitor networks does not allow us to understand the relevance of species turnover and interaction rewiring for network assembly, structure, and dynamics, nor their contribution to the maintenance of natural or modified ecosystems (Burkle et al., 2016; Poisot et al., 2015). Therefore, the aim of this study was to analyze the structure of the plant-floral visitor network and its monthly variation in a temperate forest remnant in San Miguel del Monte, Morelia, Michoacán.
The flowering phenology of the studied community occurs mainly during the rainy season and the beginning of the dry season (Cornejo-Tenorio & Ibarra-Manriquez, 2007; Cortés-Flores et al., 2015). Species turnover has been demonstrated to be strongly related to a network’s nestedness, modularity, and temporal extent, and longer sampling periods may result in reduced nestedness and increased modularity (Schwarz et al., 2020). Additionally, a recent review on the reliability of previously used methods for estimating species turnover and interaction rewiring, 2 main components in the contribution to network dissimilarity (Fründ, 2021), suggests interaction rewiring may have been overestimated. Given the temporal scale of this study and the flowering patterns described for Neotropical temperate forests (Cornejo-Tenorio & Ibarra-Manríquez, 2007; Cortés-Flores et al., 2015), the constant addition of angiosperm species to our system should favor the establishment of new interactions throughout the rainy season. Thus, we expect that the structure of our plant-floral visitor networks should be more affected by species turnover than by interaction rewiring over time. As far as we know, this is one of the first studies on the temporal variation of plant-floral visitor networks for Neotropical temperate forest ecosystems.
Materials and methods
The study was carried out in the community of San Miguel del Monte, Morelia, Michoacán, at an altitude of 2,200 m asl. The area is characterized by a mountainous relief of volcanic origin belonging to the Trans-Mexican Volcanic Belt. The predominant vegetation is pine-oak forest (Pinus teocote, P. devoniana, P. leiophylla, Quercus rugosa, Q. laurina, Q. castanea) (Franch-Pardo & Cancer-Pomar, 2017). It is worth mentioning that anthropization levels have increased considerably in recent years due to illegal burning and logging for the extensive cultivation of avocado (Persea americana). To a lesser extent, the study site has also been modified through soil extraction. Therefore, the study site corresponds to the classification of a peri-urban area undergoing severe degradation (Franch-Pardo & Cancer-Pomar, 2017).
We selected an area of 20 × 50 m and considered all flowering plants found within this area. The plant species were collected and photographed for later identification using taxonomic guides (Medina, 2000). For each plant species, we randomly selected and tagged from 5 to 10 individuals (depending on availability) and recorded the beginning, peak, and end of flowering time, as well as an estimation of the total number of flowers.
To analyze plant-floral visitor interactions, we sampled twice per month from September 2020 to March 2021 (except for March, when only 1 sampling was performed). For each plant species, we randomly selected from 1 to 10 plants (depending on their availability) and conducted direct observations in 15-20 minutes periods between 10:00 and 15:00, as it was the period with more flower visitation activity (Tavera, 2021). We recorded the identity of each floral visitor and the number of legitimate floral visits (i.e., visits in which animals contact both anthers and stigma). To determine the taxonomic identity of floral visitors, all unknown morphospecies were collected and subsequently identified to the lowest possible taxonomic level. Identification was conducted using taxonomic guides (Asher & Pickering, 2013; Michener, 2000; Triplehorn & Johnson, 2005).
To build the interaction networks, we constructed interaction matrices using the visit frequency of each floral visitor for each plant species. We calculated network metrics such as specialization, nestedness, weighted nestedness, connectance, interaction evenness, generality and vulnerability, and modularity. The values of network specialization (H2’) range from 0 (no specialization) to 1 (total specialization). This index is comparable between different networks because it is not affected by the sampling effort or network size (Kaiser-Bunbury & Blüthgen, 2015). Nestedness (NODF) indicates the qualitative nestedness of the network by the presence or absence of interactions (pattern of asymmetry in the specialization of network interactions, in which generalist taxa interact with generalist and specialist species). Weighted Nestedness (WNODF) represents the presence/absence of interactions and their frequency, comparing them to a null model in which the interaction probabilities are similar for all species. Both qualitative and weighted nestedness metrics take values between 0 (no nestedness) and 100 (perfect nestedness) (Almeida-Neto & Ulrich, 2011). Connectance is the proportion of interactions out of the total possible interactions, indicating the level of network connection (Jordano et al., 2009). Interaction evenness is a quantitative measure that indicates the magnitude of the homogeneity of interaction frequency. Its values range from 0 to 1; increasing values indicate uniformity in the distribution of the interactions (de Santiago-Hernández et al., 2019; Kaiser-Bunbury & Blüthgen, 2015). Generality and vulnerability indicate the average number of interacting partners per floral visitor and plant species (Bersier et al., 2002); when these values approach 0 they indicate high specialization. Modularity indicates whether a network is structured in modules, namely sets of species interacting more strongly with each other than with the rest of the community. Higher modularity values indicate a greater modular structure and stronger interactions within-modules (Dormann & Strauss, 2014; Kaiser-Bunbury & Blüthgen, 2015). To determine whether the network metrics observed were the result of chance, we compared each network metric with 1000 network metrics obtained from a null model. We used the null model r2dtable that keeps the sum of the rows and columns constant (R package version 4.2.1) (Dormann et al., 2009). The network metrics were considered significant when they were higher than null network metrics in 95% of comparisons. The graphical representation of the networks was performed using the Cytoscape software —version 3.8.2 (Shannon et al., 2003). To compare monthly interaction networks, we estimated the Whittaker dissimilarity index (βST/βWN) (Poisot et al., 2012). We calculated 2 components of Beta diversity (βWN): species turnover (βST) and interaction rewiring (βos). Also, we calculated the relative contribution of compositional differences in percentage given by (βST/βWN) × 100. The dissimilarity index ranges between 0 and 100, where βST/βWN < 50% indicates a low turnover of species and a greater influence of species rewiring, and βST/βWN > 50% indicates a high turnover of species, reflecting large differences in the species composition and interactions among networks. All calculations for the dissimilarity index were conducted with the “betalink” function in the “bipartite” R package (version 4.2.1) (Dormann et al., 2008, 2009; Oksanen et al., 2013).
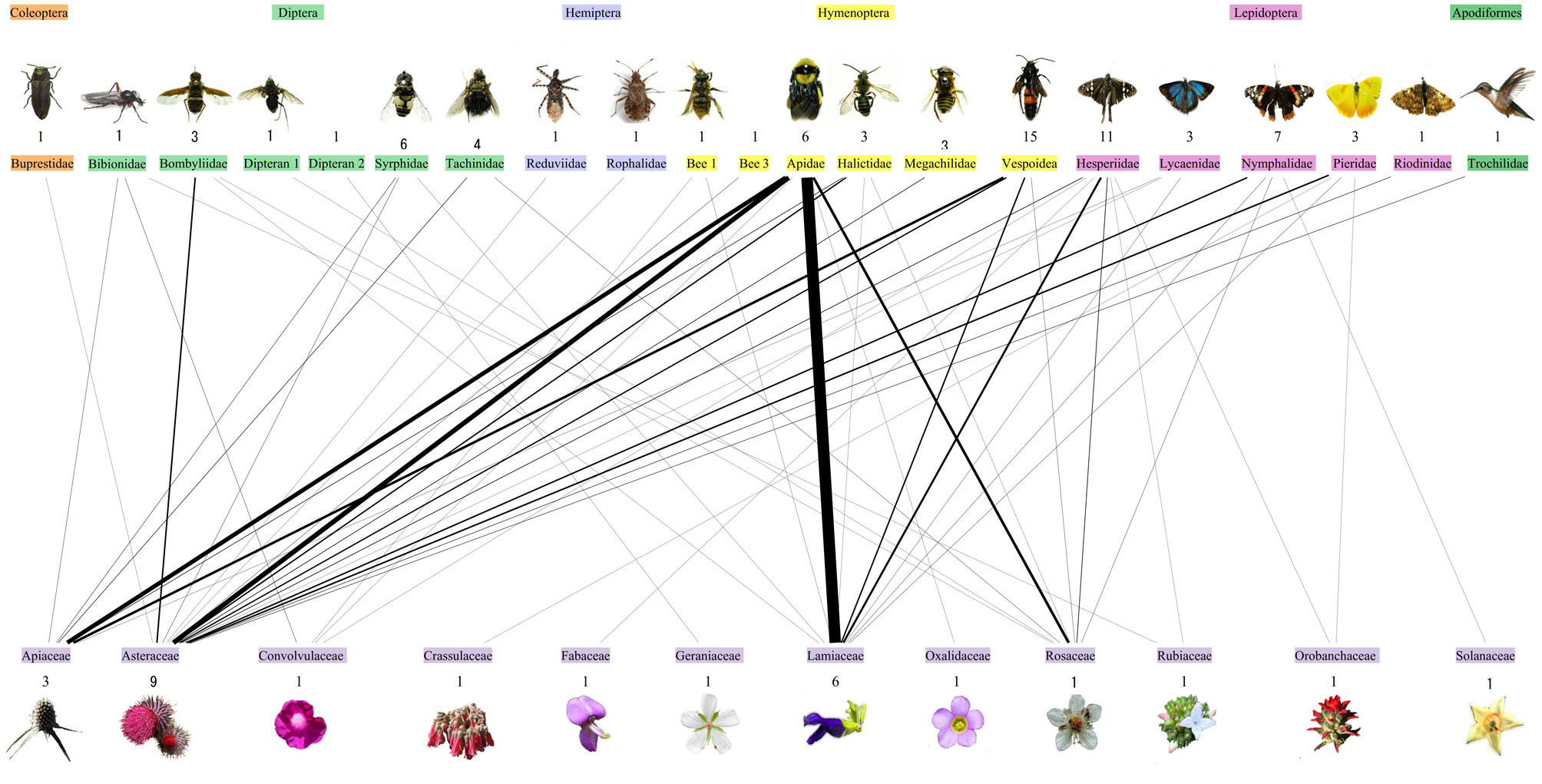
Results
We recorded a total of 45 species of angiosperms belonging to 20 plant families but only observe interactions with floral visitors in 27 of the species (Table 1). The plant families with the highest number of species were Asteraceae, 11 species, and Lamiaceae, 8 species. Three orders of insects were the most frequent floral visitors: Hymenoptera with 29 species (39.1%); Lepidoptera with 25 species (33.7%), and Diptera with 16 species (21.6%) (Fig. 1).
We recorded a total of 1,325 interactions, which included 131 exclusive one-to-one interactions. These interactions occurred between 27 angiosperm species and 74 floral visitor species (Supplementary material: Table S1). The most visited plant families were Lamiaceae with 496 interactions (30 one-to-one interactions), Asteraceae with 434 interactions (50 one-to-one interactions), and Apiaceae with 255 interactions (24 one-to-one interactions) (Supplementary material: Table S1).
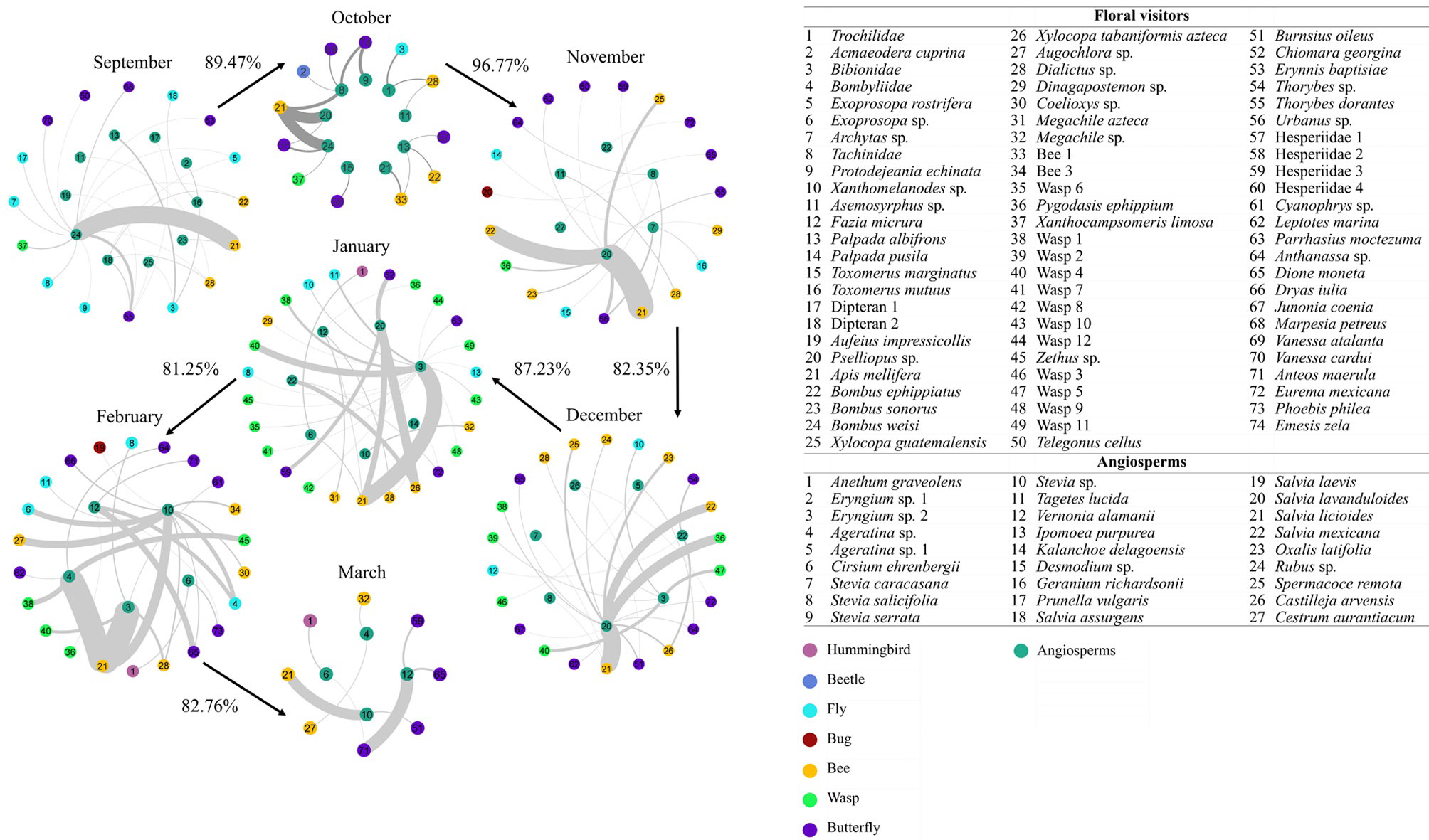
The number of plant and floral visitor species per month was highly variable. September and October were months with more flowering plant species than floral visitor species. For the rest of the months, the number of floral visitor species was higher than the number of flowering plant species. From November to February the number of plant species was reduced sequentially by ~ 50% compared to September and October, while the number of floral visitor species doubled in the same period (Fig. 2, Table 2).
The network size was similar between all monthly networks, despite the change in the number of plant and floral visitor species 27 ≥ S ≤ 36, except in March, which had the smallest network (S = 12). The network weight increased from October to February in 21%. For March, network weight was reduced by 23% concerning all networks. The number of links was similar between networks except in March, which had the network with the lowest number of links (Table 2). Specialization was generally high for all networks, where the September network had the lowest value (0.55) and the March network had the highest value (0.99). For all networks, both nestedness (NODF) and weighted nestedness (WNODF) were significant but with low values. Connectance values were low for all networks (< 0.3). February and March networks had the highest connectance values (> 0.20) (Table 2). Interaction evenness was similar for all networks. Modularity was significant for all networks, but the months with the highest values were October and March. The number of modules was similar between all networks. The null model analysis indicated that all network metrics differed significantly from zero, indicating that none of the network metric values were the result of chance (Table 2).
The results of the network dissimilarity analysis (βWN) indicated that consecutive monthly networks were highly dissimilar, with values of βWN ranging from 0.71 to 0.86. The results also indicated that network dissimilarity between consecutive months was mainly due to species turnover (βST) and not to interaction rewiring (βOS), with values of βST and βOS ranging from 0.58 to 0.77, and from 0.03 to 0.15, respectively (Table 3). Finally, the values of the Whittaker dissimilarity index (βST/ βWN) expressed as a percentage were high for all monthly network comparisons (> 80%), indicating a greater influence of species turnover to the observed dissimilarity between monthly plant-floral visitor networks (Fig. 2).
Table 1
Plant species recorded in the study site at San Miguel del Monte, Morelia, Mexico.
Family | Species name |
Amaryllidaceae | *Allium glandulosum |
Apiaceae | Anethum graveolens, Eryngium sp. 1, E. sp. 2 |
Apocynaceae | *Asclepia sp. |
Asteraceae | Ageratina sp., A. sp. 1, *A. sp. 2, Cirsium ehrenbergii, *Cosmos scabisoides, *Psacalium decompositum, Stevia salicifolia, S. serrata, S. caracasana, S. sp., Tagetes lucida, Vernonia alamanii |
Begoniaceae | *Begonia gracilis |
Caprifoliaceae | *Valeriana urticifolia |
Commelinaceae | *Commelina coelestis, *Tradescantia pinetorum |
Convolvulaceae | Ipomoea purpurea |
Crassulaceae | Kalanchoe delagoensis |
Fabaceae | Desmodium sp. |
Geraniaceae | Geranium richardsonii |
Lamiaceae | *Lepechinia caulescens, Prunella vulgaris, Salvia assurgens, *S. dichlamys, S. laevis, S. lavanduloides, S. licioides, S. mexicana |
Loganiaceae | *Spigelia scabrella |
Lythraceae | *Cuphea jorullensis |
Malvaceae | *Sida acuta |
Onagraceae | *Lopezia racemosa |
Orobanchaceae | Castilleja arvensis |
Oxalidaceae | Oxalis latifolia |
Rosaceae | Rubus sp. |
Rubiaceae | *Crusea longiflora, Spermacoce remota |
Solanaceae | *Cestrum sp., C. aurantiacum, *Physalis sp. |
The asterisk indicates species with no floral visits recorded.
Table 2
Metric values of the monthly interaction networks.
Metric | September | October | November | December | January | February | March |
Floral visitor species (A) | 16 | 12 | 19 | 22 | 26 | 22 | 8 |
Plant species (P) | 20 | 22 | 11 | 9 | 8 | 5 | 4 |
Network size (S) | 36 | 34 | 30 | 31 | 34 | 27 | 12 |
Network weight (I) | 119 | 81 | 206 | 215 | 236 | 379 | 89 |
Links (Id) | 27 | 18 | 21 | 28 | 32 | 28 | 9 |
Network specialization (H2’) | 0.55* | 0.73* | 0.73* | 0.84* | 0.63* | 0.73* | 0.99* |
Nestedness (NODF) | 20.65* | 5.16* | 9.49* | 20.16* | 13.10* | 20.12* | 10.29* |
Weighted nestedness (WNODF) | 9.17* | 3.03* | 9.05* | 10.86* | 7.22* | 13.69* | 7.35* |
Connectance (C) | 0.17* | 0.17* | 0.18* | 0.18* | 0.18* | 0.25* | 0.28* |
Interaction evenness | 0.64* | 0.81* | 0.61* | 0.76* | 0.77* | 0.79* | 0.70* |
Generality (floral visitors) | 1.58 | 2.21 | 1.15 | 1.16 | 1.64 | 2.02 | 1.06 |
Vulnerability (plants) | 3.52 | 1.86 | 3.94 | 5.92 | 4.51 | 4.01 | 1.80 |
Modularity (Q) | 0.30* | 0.51* | 0.17* | 0.36* | 0.46* | 0.41* | 0.55* |
Modules | 4 | 5 | 6 | 5 | 5 | 5 | 4 |
Links between modules | 5 | 1 | 2 | 4 | 4 | 6 | 1 |
Links within modules | 22 | 17 | 19 | 24 | 28 | 22 | 8 |
The asterisk indicates significant values.
Discussion
This study is one of the first to analyze the temporal dynamics of plant-floral visitor interactions in a pine-oak forest, and our results indicate that the number of flowering plants and floral visitor species varied widely over time. For example, in September and October networks, the number of floral visitor species was lower than that of plant species, while an opposite pattern was observed for the rest of the months. For plant species, richness decreased gradually from September to March (Supplementary material: Fig. S1). This pattern in plant species richness over time may be associated with the end of the rainy season (September and October), when most of the plant species flower and reproduce in Neotropical temperate forests (Cornejo-Tenorio & Ibarra-Manriquez, 2007; Cortés-Flores et al., 2013). After this time, from December to March, few plant species can tolerate the low winter temperatures and hydric stress, and even fewer species may flower. For floral visitors, September, October, and March were the months with the lowest number of species, and November and February were the months with the highest number of species. Most of the studies comparing the temporal variation of plant-floral visitor interaction networks have been carried out in tropical dry or rainy forests (Cortés-Flores et al., 2023; Cuartas-Hernandez & Medel, 2015; de Santiago et al., 2019; Souza et al., 2018). The absence of floral visitors in networks over time may result from temporal decoupling between insect-life cycles and flowering phenology, or migratory movements in the case of hummingbirds, or bats (Crenna et al., 2017; Morales-Garza et al., 2007; López-Segoviano et al., 2018). However, we have no evidence to support these hypotheses.
Our results indicate that the monthly plant-floral visitor networks were modular, highly specialized, and poorly connected, similar to those reported in other temperate forest studies that analyzed small plant-pollinator/ floral visitor interaction networks (Jordano et al., 2009). Our results also showed that floral visitors were highly selective when visiting plants. For example, butterflies mostly visited Asteraceae species, while flies visited plants from Apiaceae, Asteraceae, Rosaceae, and Lamiaceae, and bumblebees frequently visited plants from Lamiaceae, Apiaceae, Asteraceae, and Rosaceae. The selectivity of floral visitors towards certain plant species increased specialization from September to March. Specifically, March (dry season) was the month when the network had the highest specialization. The evidence suggests that in most ecosystems, reduction in flowering plant and floral visitor diversity is influenced by water stress and extreme temperatures that affect the survival of plants and floral visitors (Argueta-Guzmán et al., 2022; Basilio et al., 2006; Bawa et al., 2003; Borchert et al., 2004; Cortés-Flores et al., 2017, 2023; Escobedo-Kenefic et al., 2020; Petanidou et al., 2018; Rabeling et al., 2019; Ramírez, 2006; Robinson et al., 2018). This reduction in floral visitors and flowering plant species during the dry season promotes specialized pollination/ floral visitation networks (Cortés-Flores et al., 2023; Souza et al., 2018), like those observed for temperate forests in Argentina (Basilio et al., 2006) and Guatemala (Escobedo-Kenefic et al., 2020). Therefore, the gradual reduction of both plant and floral visitor diversity, as well as the increasing network specialization observed in our study, resulted from the environmental conditions of the dry season.
Table 3.
β diversity and its 2 main components between consecutive monthly interaction networks.
β diversity 2 main components | |||
Consecutive months | β diversity βWN | Species turnover
βST |
Interaction rewiring
βOS |
Sept – Oct | 0.86 | 0.77 | 0.09 |
Oct – Nov | 0.79 | 0.77 | 0.03 |
Nov – Dec | 0.71 | 0.58 | 0.13 |
Dec – Jan | 0.8 | 0.69 | 0.1 |
Jan – Feb | 0.8 | 0.65 | 0.15 |
Feb – Mar | 0.78 | 0.65 | 0.14 |
Although our networks were highly specialized, the nestedness (NODF) and weighted nestedness (WNODF) analyses were significant, indicating that in all monthly networks, there were plant and floral visitor species interacting simultaneously with generalist and specialist plant species. Although both qualitative (NODF) and quantitative (WNODF) nestedness were significant, network specialization promoted low nestedness values (Almeida-Neto & Ulrich, 2011; Almeida-Neto et al., 2008). For example, February was the most nested network (NODF and WNODF), when Stevia sp. interacted with 11 of 22 floral visitor species, and Apis mellifera interacted with 3 of 5 plant species. At the same time, 12 floral visitor species had unique interactions with a single plant species, and 1 plant species had unique interactions with 2 floral visitor species. Therefore, although nestedness was significant in all networks, many interactions were specialized within each interaction network, suggesting that the interpretation of nestedness in terms of significance and metric values should be taken carefully as other studies have suggested (i.e., Cook & Quinn, 1998; Gotelli, 2001; Fischer & Lindenmayer, 2002). Likewise, Ramos-Jiliberto (2009), Spiesman and Inouye (2013) and Escobedo-Kenefic et al. (2020) report an overall nested structure in their floral-visitation networks, though only Escobedo-Keneffic et al. (2020) analyzed temporal variation, and associate higher nestedness with the dry season.
When analyzing monthly networks modularity, January, October, and March networks had the highest modularity values and showed the most specialized networks, while September, November, and December networks were the least modular and least specialized. Some studies suggest that high modularity indicates strong within-module interactions, which reflects more specialization, and low modularity indicates that plant-floral visitor interactions are more generalist (Dormmand et al., 2012, 2014). In our study, the number of modules was not different between networks, but the intra-module interacting plant and floral visitor species assemblages differed between monthly networks. Contrary to our results, where no clear pattern in modularity can be easily observed, some other studies have found a positive or a negative relation between network species richness and modularity (Escobedo-Kenefic et al., 2020; Spiesman & Inouye, 2013).
Similar to other studies, we found that the modules are composed of a few plant species interacting with a greater number of floral visitor species (Dupont & Olesen, 2009). It has been suggested that modules in networks based on pollinator/ floral visitor frequency are composed of legitimate pollinators and frequent floral visitors (de Santiago-Hernández et al., 2019). Hence, it is important to highlight that our frequency-based network modules showed associations between some specific plant and floral visitor species. For example, for all networks, plants belonging to the genus Eryngium formed a module with wasp and fly species; likewise, plants from the Asteraceae family formed modules with butterfly species.
When we analyzed the dissimilarity between monthly networks, the difference between networks was explained by species turnover, reaching values of 97% between consecutive months (Fig. 2). Our results indicate that flowering plant species richness decreased gradually from September to March as winter and dry seasons arrived (Cornejo-Tenorio & Ibarra-Manríquez, 2007; Cortés-Flores et al., 2013). Therefore, the floral phenology of plant species is the most reasonable driver for changes in the interaction network’s structure between months in our study.
The low contribution of interaction rewiring to network dissimilarity was due to few plant and floral visitor species shared between consecutive monthly networks. For example, Apis mellifera was the only floral visitor present in all monthly networks, while no plant species flowering season lasted for the whole sampling period. Salvia lavanduloides and Eryngium sp. 2 were the plant species with the longest floral phenology, from October to January and from December to February, respectively (Table 4). In the case of S. lavanduloides, it interacted mostly with A. mellifera, while Eryngium sp. 2 interacted mostly with wasp species and A. mellifera (Fig. 1). These results suggest that although some networks shared plant and floral visitor species, the interactions between them remained constant. Therefore, interaction rewiring did not contribute significantly to network dissimilarity. Similarly, Basilio et al. (2006) observed low average similarity between monthly floral-visitation networks, and only few plant and floral visitor species involved in many interactions and present through the whole sampling period.
It is important to note that the plant species differed in abundance and consequently in number of flowers at the study site. Abundant plant species with many flowers are visited more often than less abundant species with fewer flowers (Goulson, 1999; Hegland & Boeke, 2006; Hernández-Villa et al., 2020). For example, Salvia lavanduloides and Eryngium sp. 2 were the most abundant plant species, produced many flowers, and had many interactions during their flowering period compared to Stevia sp., which was less abundant and received very few visits. For floral visitors, a higher number of visits was associated with their abundance. For example, A. mellifera was the most abundant in all networks given its social behavior. In addition, A. mellifera is a generalist bee species, which may displace or reduce the visitation frequency of other floral visitors (Valido et al., 2019). In this way, A. mellifera may alter the interaction networks between native plants and pollinators (Aizen et al., 2008; de M. Santos et al., 2012). It is unknown to what extent A. mellifera may modify the dynamics of the ecosystems to which it has been introduced, so more studies are needed to evaluate its effect on native plant-pollinator communities.
Finally, pine-oak forest plant-floral visitor interaction networks are very dynamic, with low connectivity, and high species turnover. These features of plant-floral visitor interactions suggest that pine-oak forests are vulnerable to drastic environmental changes (Spiesman & Inouye, 2013). Therefore, plant-floral visitor interaction networks are constantly threatened due to continuous land-use changes and global warming related effects that compromise their long-term permanence and functionality.
Acknowledgments
The authors thank the Associate Editor and two anonymous reviewers for their insightful suggestions on early versions of the manuscript.
References
Aizen, M. A., Morales, C. L., & Morales, J. M. (2008). Invasive mutualists erode native pollination webs. Plos Biology, 6, e31. https://doi.org/10.1371/journal.pbio.0060031
Alarcón, R., Waser, N. M., & Ollerton, J. (2008). Year-to-year variation in the topology of a plant–pollinator interaction network. Oikos, 117, 1796–1807. https://doi.org/
10.1111/j.0030-1299.2008.16987.x
Almeida-Neto, M., Guimaraes, P., Guimaraes, P. R. Jr., Loyola, R. D., & Ulrich, W. (2008). A consistent metric for nestedness analysis in ecological systems: reconciling concept and measurement. Oikos, 117, 1227–1239. https://doi.org/10.1111/j.0030-1299.2008.16644.x
Almeida-Neto, M., & Ulrich, W. (2011). A straightforward computational approach for measuring nestedness using quantitative matrices. Environmental Modelling y Software, 26, 173–178. https://doi.org/10.1016/j.envsoft.2010.08.003
Argueta-Guzmán, M., Golubov, J., Cano-Santana, Z., & Ayala, R. (2022). The role of seasonality and disturbance in bee–plant interactions in semi-arid communities of the southern Chihuahuan desert. Insect Conservation and Diversity, 15, 543–554. https://doi.org/10.1111/icad.12572
Ascher, J. S., & Pickering, J. (2013). Discover life bee species guide and world checklist (Hymenoptera: Apoidea: Anthophila). Available at: https://www.discoverlife.org/mp/20q?guide=Apoidea_species&flags=HAS:
Baldock, K. C., Memmott, J., Ruiz-Guajardo, J. C., Roze, D., & Stone, G. N. (2011). Daily temporal structure in African savanna flower visitation networks and consequences for network sampling. Ecology, 92, 687–698. https://doi.org/
10.1890/10-1110.1
Bascompte, J., Jordano, P., Melián, C. J., & Olesen, J. M. (2003). The nested assembly of plant–animal mutualistic networks. Proceedings of the National Academy of Sciences, 100, 9383–9387. https://doi.org/10.1073/pnas.1633576100
Basilio, A. M., Medan, D., Torretta, J. P., & Bartoloni, N. J. (2006). A year-long plant-pollinator network. Austral Ecology, 31, 975–983. https://doi.org/10.1111/j.1442-9993.2006.01666.x
Bawa, K. S., Kang, H., & Grayum, M. H. (2003). Relationships among time, frequency, and duration of flowering in tropical rain forest trees. American Journal of Botany, 90, 877–887. https://doi.org/10.3732/ajb.90.6.877
Bersier, L. F., Banašek-Richter, C., & Cattin, M. F. (2002).
Quantitative descriptors of food-web matrices. Ecology,
83, 2394–2407. https://doi.org/10.1890/0012-9658(2002)083
[2394:QDOFWM]2.0.CO;2
Blüthgen, N., Fründ, J., Vázquez, D. P., & Menzel, F. (2008). What do interaction network metrics tell us about specialization and biological traits. Ecology, 89, 3387–3399. https://doi.org/10.1890/07-2121.1
Borchert, R., Meyer, S. A., Felger, R. S., & Porter-Bolland, L. (2004). Environmental control of flowering periodicity in Costa Rican and Mexican tropical dry forests. Global Ecology and Biogeography, 13, 409–425. https://doi.org/10.1111/j.1466-822X.2004.00111.x
Burkle, L., & Irwin, R. (2009). The importance of interannual variation and bottom–up nitrogen enrichment for plant–pollinator networks. Oikos, 118, 1816–1829. https://doi.org/10.1111/j.1600-0706.2009.17740.x
Burkle, L. A., Marlin, J. C., & Knight, T. M. (2013). Plant-pollinator interactions over 120 years: loss of species, co-occurrence, and function. Science, 339, 1611–1615. https://doi.org/10.1126/science.1232728
Burkle, L. A., Myers, J. A., & Belote, R. T. (2016). The beta-diversity of species interactions: Untangling the drivers of geographic variation in plant–pollinator diversity and function across scales. American Journal of Botany, 103, 118–128. https://doi.org/10.3732/ajb.1500079
CaraDonna, P. J., Petry, W. K., Brennan, R. M., Cunningham, J. L., Bronstein, J. L., Waser, N. M. et al. (2017). Interaction rewiring and the rapid turnover of plant–pollinator networks. Ecology Letters, 20, 385–394. https://doi.org/10.1111/ele.
12740
Cook, R. R., & Quinn, J. F. (1998). An evaluation of randomization models for nested species subsets analysis. Oecologia, 113, 584–592. https://doi.org/10.1007/s004420050412
Cornejo-Tenorio, G., & Ibarra-Manríquez, G. (2007). Plant reproductive phenology in a temperate forest of the Monarch Butterfly Biosphere Reserve, Mexico. Interciencia, 32, 445–452.
Cortés-Flores, J., Andresen, E., Cornejo-Tenorio, G., & Ibarra-Manríquez, G. (2013). Fruiting phenology of seed dispersal syndromes in a Mexican Neotropical temperate forest. Forest Ecology and Management, 289, 445–454. https://doi.org/10.1016/j.foreco.2012.10.038
Cortés-Flores, J., Cornejo-Tenorio, G., & Ibarra-Manríquez, G. (2015). Flowering phenology and pollination syndromes in species with different growth forms in a Neotropical temperate forest of Mexico. Botany, 93, 361–367. https://doi.org/10.1139/cjb-2014-0218
Cortés-Flores, J., Hernández-Esquivel, K. B., González-Rodríguez, A., & Ibarra-Manríquez, G. (2017). Flowering phenology, growth forms, and pollination syndromes in tropical dry forest species: Influence of phylogeny and abiotic factors. American Journal of Botany, 104, 39–49. https://doi.org/10.3732/ajb.1600305
Cortés-Flores, J., Lopezaraiza-Mikel, M., de Santiago-Hernández, M. H., Martén-Rodríguez, S., Cristóbal-Pérez, E. J., Aguilar-Aguilar, M. J. et al. (2023). Successional and phenological effects on plant-floral visitor interaction networks of a tropical dry forest. Journal of Ecology, 111, 927–942. https://doi.org/10.1111/1365-2745.14072
Crenna, E., Sala, S., Polce, C., & Collina, E. (2017). Pollinators in life cycle assessment: towards a framework for impact assessment. Journal of Cleaner Production, 140, 525–536. https://doi.org/10.1016/j.jclepro.2016.02.058
Cuartas-Hernández, S., & Medel, R. (2015). Topology of plant-flower-visitor networks in a tropical mountain forest: insights on the role of altitudinal and temporal variation. Plos One, 10, e0141804. https://doi.org/10.1371/journal.pone.0141804
de M. Santos, G. M., Aguiar, C. M., Genini, J., Martins, C. F., Zanella, F. C., & Mello, M. A. (2012). Invasive Africanized honeybees change the structure of native pollination networks in Brazil. Biological Invasions, 14, 2369–2378. https://doi.org/10.1007/s10530-012-0235-8
de Santiago-Hernández, M. H., Martén-Rodríguez, S., Lopezaraiza-Mikel, M., Oyama, K., González-Rodríguez, A., & Quesada, M. (2019). The role of pollination effectiveness on the attributes of interaction networks: from floral visitation to plant fitness. Ecology, 100, e02803. https://doi.org/10.1002/ecy.2803
Dormann, C. F., Fründ, J., Blüthgen, N., & Gruber, B. (2009). Indices, Graphs and Null Models: Analyzing Bipartite Ecological Networks. The Open Ecology Journal, 2, 7-24.
Dormann, C. F., Gruber, B., & Fründ, J. (2008). Introducing the bipartite package: analyzing ecological networks. Interaction, 1, 0.2413793.
Dormann, C. F., & Strauss, R. (2014). A method for detecting modules in quantitative bipartite networks. Methods in Ecology and Evolution, 5, 90–98. https://doi.org/10.1111/2041-210X.12139
Dupont, Y. L., & Olesen, J. M. (2009). Ecological modules and roles of species in heathland plant-insect flower visitor networks. Journal of Animal Ecology, 78, 346–353.
Dupont, Y. L., Padrón, B., Olesen, J. M., & Petanidou, T. (2009). Spatio-temporal variation in the structure of pollination networks. Oikos, 118, 1261–1269. https://doi.org/10.1111/j.1600-0706.2009.17594.x
Escobedo-Kenefic, N., Landaverde-González, P., Theodorou, P., Cardona, E., Dardón, M. J., Martínez, O. et al. (2020). Disentangling the effects of local resources, landscape heterogeneity and climatic seasonality on bee diversity and plant-pollinator networks in tropical highlands. Oecologia, 194, 333–344. https://doi.org/10.1007/s00442-020-04715-8
Fischer, J., & Lindenmayer, D. B. (2002). Treating the nestedness temperature calculator as a black box can lead to false conclusions. Oikos, 99, 193–199. https://doi.org/10.1034/j.1600-0706.2002.990121.x
Franch-Pardo, I., & Cancer-Pomar, L. (2017). El componente visual en la cartografía del paisaje. Aptitud paisajística para la protección en la cuenca del río Chiquito (Morelia, Michoacán). Investigaciones Geográficas, Boletín del Instituto de Geografía, 93, 42–60. https://doi.org/10.14350/rig.54730
Fründ, J. (2021). Dissimilarity of species interaction networks: how to partition rewiring and species turnover components. Ecosphere, 12, e03653. https://doi.org/10.1002/ecs2.3653
Gaston, K. J. (1996). Biodiversity-latitudinal gradients. Progress in Physical Geography, 20, 466–476. https://doi.org/10.1177/030913339602000406
Gotelli, N. J. (2001). Research frontiers in null model analysis. Global Ecology and Biogeography, 10, 337–343. https://doi.org/10.1046/j.1466-822X.2001.00249.x
Goulson, D. (1999). Foraging strategies of insects for gathering nectar and pollen, and implications for plant ecology and evolution. Perspectives in Plant Ecology, Evolution and Systematics, 2, 185–209. https://doi.org/10.
1078/1433-8319-00070
Guzman, L. M., Chamberlain, S. A., & Elle, E. (2021). Network robustness and structure depend on the phenological characteristics of plants and pollinators. Ecology and Evolution, 11, 13321–13334. https://doi.org/10.1002/ece3.
8055
Hegland, S. J., & Boeke, L. (2006). Relationships between the density and diversity of floral resources and flower visitor activity in a temperate grassland community. Ecological Entomology, 31, 532–538. https://doi.org/10.11
11/j.1365-2311.2006.00812.x
Hernández-Villa, V., Vibrans, H., Uscanga-Mortera, E., & Aguirre-Jaimes, A. (2020). Floral visitors and pollinator dependence are related to floral display size and plant height in native weeds of central Mexico. Flora, 262, 151505. https://doi.org/10.1016/j.flora.2019.151505
Jordano, P. (2016). Sampling networks of ecological interactions. Functional Ecology, 30, 1883–1893. https://doi.org/10.1111/1365-2435.12763
Jordano, P., Bascompte, J., & Olesen, J. M. (2003). Invariant properties in coevolutionary networks of plant–animal interactions. Ecology Letters, 6, 69–81. https://doi.org/10.
1046/j.1461-0248.2003.00403.x
Jordano, P., Vázquez, D., & Bascompte, J. (2009). Redes complejas de interacciones planta-animal. En R. Medel, M. A. Aizen, & R. Zamora (Eds.), Ecología y evolución de interacciones planta-animal (pp. 17–41). Santiago, Chile: Editorial Universitaria.
Kaiser-Bunbury, C. N., & Blüthgen, N. (2015). Integrating network ecology with applied conservation: a synthesis and guide to implementation. AoB Plants, 7, 1–15. https://doi.org/10.1093/aobpla/plv076
Kevan, P. G., & Baker, H. G. (1983). Insects as flower visitors and pollinators. Annual review of entomology, 28, 407–453. https://doi.org/10.1146/annurev.en.28.010183.002203
López-Segoviano, G., Arenas-Navarro, M., Vega, E., & Arizmendi, M. C. (2018). Hummingbird migration and flowering synchrony in the temperate forests of north-
western Mexico. PeerJ, 6, e5131. https://doi.org/10.7717/peerj.5131
Medina, C. (2000). Flora del río chiquito, Morelia, Michoacán, México. Chicago: Environmental and Conservation Programs.
Michener, C. D. (2000). The bees of the world. Baltimore, MD: JHU Press.
Morales-Garza, M. R., Arizmendi, M. C., Campos, J. E., Martínez-García, M., & Valiente-Banuet, A. (2007). Evidences on the migratory movements of the nectar-feeding bat Leptonycteris curasoae in Mexico using random amplified polymorphic DNA (RAPD). Journal of Arid Environments, 68, 248–259. https://doi.org/10.1016/j.jaridenv.2006.05.009
Morente-López, J., Lara-Romero, C., Ornosa, C., & Iriondo, J. M. (2018). Phenology drives species interactions and modularity in a plant-flower visitor network. Scientific Reports, 8, 1–11. https://doi.org/10.1038/s41598-018-27725-2
Oksanen, J., Blanchet, F. G., Kindt, R., Legendre, P., Minchin, P. R., O’hara, R. B. et al. (2013). Package ‘vegan’. Community Ecology Package, Version, 2, 1–295.
Olesen, J. M., Bascompte, J., Dupont, Y. L., Elberling, H., Rasmussen, C., & Jordano, P. (2011). Missing and forbidden links in mutualistic networks. Proceedings of the Royal Society B: Biological Sciences, 278, 725–732. https://doi.org/10.1098/rspb.2010.1371
Olesen, J. M., Bascompte, J., Dupont, Y. L., & Jordano, P. (2007). The modularity of pollination networks. Proceedings of the National Academy of Sciences, 104, 19891–19896. https://doi.org/10.1073/pnas.0706375104
Olesen, J. M., & Jordano, P. (2002). Geographic patterns in plant–pollinator mutualistic networks. Ecology, 83, 2416–2424. https://doi.org/10.1890/0012-9658(2002)083[2416:GPIPPM]2.0.CO;2
Ollerton, J., Winfree, R., & Tarrant, S. (2011). How many flowering plants are pollinated by animals? Oikos, 120, 321–326. https://doi.org/10.1111/j.1600-0706.2010.18644.x
Petanidou, T., Kallimanis, A. S., Lazarina, M., Tscheulin, T., Devalez, J., Stefanaki, A. et al. (2018). Climate drives plant–pollinator interactions even along small-scale climate gradients: the case of the Aegean. Plant Biology, 20, 176–183. https://doi.org/10.1111/plb.12593
Petanidou, T., Kallimanis, A. S., Tzanopoulos, J., Sgardelis, S. P., & Pantis, J. D. (2008). Long-term observation of a pollination network: fluctuation in species and interactions, relative invariance of network structure and implications for estimates of specialization. Ecology Letters, 11, 564–575. https://doi.org/10.1111/j.1461-0248.2008.01170.x
Poisot, T. (2022). Dissimilarity of species interaction networks: quantifying the effect of turnover and rewiring. Peer Community Journal, 2, 335. https://doi.org/10.24072/pc
journal.105
Poisot, T., Canard, E., Mouillot, D., Mouquet, N., & Gravel, D. (2012). The dissimilarity of species interaction networks. Ecology Letters, 15, 1353–1361. https://doi.org/10.24072/pcjournal.105
Poisot, T., Stouffer, D. B., & Gravel, D. (2015). Beyond species: why ecological interaction networks vary through space and time. Oikos, 124, 243–251. https://doi.org/10.1111/oik.01719
Rabeling, S. C., Lim, J. L., Tidon, R., Neff, J. L., Simpson, B. B., & Pawar, S. (2019). Seasonal variation of a plant-pollinator network in the Brazilian Cerrado: implications for community structure and robustness. Plos One, 14, e0224997. https://doi.org/10.1371/journal.pone.0224997
Ramírez, N. (2006). Temporal variation of pollination classes in a tropical Venezuelan plain: the importance of habitats and life forms. Botany, 84, 443–452. https://doi.org/10.1139/b06-015
Ramos-Jiliberto, R., Albornoz, A. A., Valdovinos, F. S., Smith-Ramírez, C., Arim, M., Armesto, J. J. et al. (2009). A network analysis of plant-pollinator interactions in temperate rain forests of Chiloé Island, Chile. Oecologia, 160, 697–706. https://doi.org/10.1007/s00442-009-1344-7
Rasmussen, C., Dupont, Y. L., Mosbacher, J. B., Trøjelsgaard, K., & Olesen, J. M. (2013). Strong impact of temporal resolution on the structure of an ecological network. Plos One, 8, e81694. https://doi.org/10.1371/journal.pone.0081694
Robinson, S. V., Losapio, G., & Henry, G. H. (2018). Flower-power: Flower diversity is a stronger predictor of network structure than insect diversity in an Arctic plant–pollinator network. Ecological Complexity, 36, 1–6. https://doi.org/
10.1016/j.ecocom.2018.04.005
Rosas-Guerrero, V., Aguilar, R., Martén-Rodríguez, S., Ashworth, L., Lopezaraiza-Mikel, M., Bastida, J. M. et al. (2014). A quantitative review of pollination syndromes: do floral traits predict effective pollinators? Ecology Letters, 17, 388–400. https://doi.org/10.1111/ele.12224
Schwarz, B., Vázquez, D. P., CaraDonna, P. J., Knight, T. M., Benadi, G., Dormann, C. F. et al. (2020). Temporal scale-dependence of plant-pollinator networks. Oikos, 129, 1289–1302. https://doi.org/10.1111/oik.07303
Shannon, P., Markiel, A., Ozier, O., Baliga, N. S., Wang, J. T., Ramage, D. et al. (2003). Cytoscape: a software environment for integrated models of biomolecular interaction networks. Genome Research, 13, 2498–2504.
Soares, R. G. S., Ferreira, P. A., & Lopes, L. E. (2017). Can plant-pollinator network metrics indicate environmental quality? Ecological Indicators, 78, 361–370. https://doi.org/10.1016/j.ecolind.2017.03.037
Souza, C. S., Maruyama, P. K., Aoki, C., Sigrist, M. R., Raizer, J., Gross, C. L. et al. (2018). Temporal variation in plant–pollinator networks from seasonal tropical environments: higher specialization when resources are scarce. Journal of Ecology, 106, 2409–2420. https://doi.org/10.1111/1365-2745.12978
Spiesman, B. J., & Inouye, B. D. (2013). Habitat loss alters the architecture of plant–pollinator interaction networks. Ecology, 94, 2688–2696. https://doi.org/10.1890/13-0977.1
Tavera, A. (2021). Caracterización de la red de interacciones planta-visitante floral de la comunidad de Agua Zarca, San Miguel del Monte (Bachelor Thesis). Facultad de Biología, Universidad Michoacana de San Nicolás de Hidalgo, Morelia, México.
Traveset, A., Tur, C., Trøjelsgaard, K., Heleno, R., Castro-Urgal, R., & Olesen, J. M. (2016). Global patterns of mainland and insular pollination networks. Global Ecology and Biogeography, 25, 880–890. https://doi.org/10.1111/geb.12362
Triplehorn, A. C., & Johnson, F. N. (2005). Borror and Delong´s introduction to the study of insects, 7th edition. Florence, KY: Thomson Brooks/Cole.
Valido, A., Rodríguez-Rodríguez, M. C., & Jordano, P. (2019). Honeybees disrupt the structure and functionality of plant-pollinator networks. Scientific Reports, 9, 1–11. https://doi.org/10.1038/s41598-019-41271-5
Vizentin-Bugoni, J., Maruyama, P. K., de Souza, C. S., Ollerton, J., Rech, A. R., & Sazima, M. (2018). Plant-pollinator networks in the tropics: a review. In W. Dáttilo, & V. Rico-Gray (Eds.), Ecological networks in the tropics (pp. 73–91). Springer, Cham. https://doi.org/10.1007/978-3-319-68228-0_6
Willig, M. R., Kaufman, D. M., & Stevens, R. D. (2003). Latitudinal gradients of biodiversity: pattern, process, scale, and synthesis. Annual Review of Ecology, Evolution, and Systematics, 34, 273–309. https://doi.org/10.1146/annurev.ecolsys.34.012103.144032