Ten-year study of vegetation dynamics in wetlands subject to human disturbance in Western Mexico
Cindy Rodríguez-Arias a, Mariela Gómez-Romero b, María Elena Páramo-Pérez b, Roberto Lindig-Cisneros b, *
a Sede de Occidente de la Universidad de Costa Rica. Apartado postal 111-4250, San Ramón, Costa Rica
b Laboratorio de Restauración Ambiental, Instituto de Investigaciones en Ecosistemas y Sustentabilidad, Universidad Nacional Autónoma de México, Campus Morelia, Antigua Carretera a Pátzcuaro No. 8701, 58190 Morelia, Michoacán, Mexico
* Corresponding author: rlindig@iies.unam.mx (R. Lindig-Cisneros)
Abstract
Sustained human disturbances cause trajectory shifts in wetland plant communities and allow invasive species to colonize. Understanding long term impacts of these disturbances is necessary for better management practices and environmental restoration. In this study we evaluated species composition in a fen type wetland for 10 consecutive years subjected to intentional fires and cattle grazing. We found that fires alter spatial distribution and relative abundance of wetland species but, at least in this wetland, no species loss was recorded for the 10-year period. We did document an increment over time in the proportion of facultative hydrophytes and a decrease in the number of obligate species. Cattle browsing damaged wetland plants, but its removal allows the introduced species Festuca arundinacea to dominate areas formerly occupied by Schoenoplectus americanus. These results show the interplay of disturbances and how elimination of a single disturbance can degrade the plant community. The changing trends suggest that current species composition might not revert naturally and that active management for restoration is needed.
Keywords:
Fire; Cattle; Trajectories; Invasive species; Restoration
© 2018 Universidad Nacional Autónoma de México, Instituto de Biología. Este es un artículo Open Access bajo la licencia CC BY-NC-ND
Estudio de diez años de la dinámica de la vegetación de humedales sometidos a disturbios de origen humano en el occidente de México
Resumen
Las alteraciones sostenidas de origen humano causan cambios en la trayectoria de las comunidades de plantas de humedal y permiten que especies invasoras colonicen. Entender el impacto a largo plazo de estas alteraciones es necesario para contar con mejores prácticas de manejo y restauración ambiental. Se evaluó la composición de especies en un humedal (marjal) por 10 años consecutivos que ha sido sometido a fuegos inducidos y pastoreo. Los resultados sugieren que los incendios alteran la distribución espacial y la abundancia relativa de las especies, pero no se detectaron pérdidas de especies en el mismo período. El principal cambio fue la proporción entre hidrófitas obligadas y facultativas, las segundas volviéndose más abundantes con el paso del tiempo y eventualmente superando a las primeras. El pastoreo daña a las plantas del humedal, pero eliminarlo permite que la especie introducida Festuca arundinacea domine áreas en donde anteriormente dominaba Schoenoplectus americanus. Estos resultados muestran la interrelación entre disturbios y cómo al eliminar uno de ellos, se puede favorecer mayor degradación de la comunidad vegetal. Los patrones de cambio también sugieren que la composición actual no retorne de manera natural al estado anterior al disturbio y que es necesario un manejo para la restauración.
Palabras clave:
Fuego; Ganado; Trayectorias; Especies invasoras; Restauración
Introduction
Natural plant distribution depends on various factors that influence population dynamics and the relationships among species. Preponderant among such factors are climate, soil, and disturbance regimes which in combination determine and cause changes in plant physiognomy and cover (Brun et al., 2012). In many cases, modifications in environmental conditions often lead to directional changes in plant composition. The specific trajectories depend on factors such as species composition of the initial community, the type of perturbation, and its intensity. Consequently, changes in an environmental driver may gradually impair the resilience of the community and give rise to a particular directional change until a threshold is reached that will result in an abrupt change in species composition (Sah et al., 2014).
Wetlands stand out among plant communities because the plant species found there have special adaptations that allow them to tolerate the unique conditions of such habitats, especially hydric soils. Consequently, wetland plants are good indicators of the environmental condition of wetlands, since these plant species are easy to identify, they respond to changes in the hydroperiod, water chemistry, substrate type, landscape connectivity to seed sources, border effects, and changes in the disturbance regime (Hernández et al., 2015). Therefore, long term monitoring of vegetation is, on the one hand, technically feasible and, on the other, essential in obtaining information for adaptive management of wetland ecosystems (Sah et al., 2014).
Fire is a disturbance that is a key factor in the natural dynamics of land ecosystems such as grasslands, shrubland, savannas and boreal forests (Bond y Keleey, 2005). In contrast, fire is not frequent in wetlands due to the high soil moisture and air humidity levels and the differences in fire susceptibility when compared to upland ecosystems (Cronk & Fennesy, 2001; Maltby & Barker, 2009). Nevertheless, lightning, spontaneous combustion (Dwire & Boone-Kauffman, 2003) and spreading of upland natural or intentional fires may sometimes cause wetlands to catch fire (Miller, 1997; Pettit & Naiman, 2007). The main responses of wetlands to fire may be: an increase or decrease in the diversity of the plant community because different plant species respond differentially to fire (Gabrey & Afton, 2001; Kost & Steven, 2001), a decrease in the number of aquatic bird and insect species (Gabrey & Afton, 1999; Hochkirchi & Adorf, 2007), an increase or loss in nutrient availability, depending on fire frequency and intensity nutrients can be released from the burned organic material or volatilized (Laubhan, 1995) and an increase in primary production (Gabrey & Afton, 2001).
Low to medium intensity fires consume only a portion of the plant biomass, and in heterogeneous sites they create patches of vegetation that serve as a refuge for fire-sensitive species (Pettit & Naiman, 2007). These shelters may allow for plant recolonization. For example, gallery forests on river banks include fire-sensitive plant species that are not found in the adjacent upland ecosystems (Dwire & Boone-Kauffman, 2003; Pettit & Naiman, 2007). The presence of an expanse of water or of water saturated soils prevents heat from radiating into the soil and has an effect on fire intensity and on the way it spreads (Cronk & Fennesy, 2001) by protecting roots, rhizomes and seed banks thus allowing for rapid recovery of the existing species (Keddy, 2010). Fire alters nutrient concentrations in wetlands by the volatilization of nitrogen and the mineralization of the phosphorus contained in the ashes of the burnt plant biomass (Laubhan, 1995). Fire increases light availability by opening the canopy, which in turn increases the water temperature and has an effect also on the growth of the aquatic plants that emerge from lower strata. Moreover, microsite availability increases after fires, thus favoring the richness and diversity of wetland species (Kost & Steven, 2001). The resulting plant composition will depend on the floristic composition and abiotic factors that existed prior to the disturbance.
High intensity fires during the dry season and/or in disturbed wetlands may destroy all plants (including roots and rhizomes), most organic matter and may volatilize nutrients. In addition, fire can cause loss of soil through erosion. Following high intensity fires the ecosystem is often recolonized by opportunistic species, giving rise to impoverished plant communities (Bisson et al., 2003).
Fire also modifies the composition and structure of plant communities because the response differs among the various species. Adaptations possessed by some species that grow in flooded areas allow rapid recovery after fire (Blom & Voesenek, 1996; Carter & Grace, 1990). This rapid recovery is associated with fast growth rates that are a consequence of high photosynthetic rates (Johnson & Knapp, 1993; Sand-Jensen & Frost-Christensen, 1999). This mechanism has been reported for Typha spp. and Phragmites australis (Cav.) Trin. ex Steud in North American wetlands (Baruch & Goldstein, 1999; Wu et al., 2012).
In addition to fires, grazing in wetlands has differential effects depending on the type of wetland. In large seasonal wetlands grazing is in many areas not considered a conservation threat, in particular if it occurs seasonally and in low densities (Contini & Cannicci, 2009). But for many wetlands, especially small and isolated, the effects of grazing are usually detrimental. These effects result from 4 processes (Morris & Reich, 2013): treading, transport of plant seeds into the wetland, deposition of urine and excrement in the wetland, and herbivory. These processes can alter wetland condition by modifying water quality and regime, soil properties, by facilitating plant invasions and in general by altering wetland vegetation structure.
La Mintzita springs and wetlands, located to the south of the city of Morelia in the state of Michoacán, Mexico, are a complex system of different wetland types, including the springs that give its name to the area, and fen like wetlands that are fed by low nutrient spring water. The water flows through a rocky bottom, creating numerous and diverse habitats (Ramírez-Herrejón et al., 2013) that shelter fauna and flora. La Mintzita springs are one of the main water sources for the city of Morelia (approximately 40% of municipal potable water supply) (Gámez & Lindig-Cisneros, 2014). The fen like wetlands are subject to various forms of human disturbance, among which fire stands out (Escutia-Lara et al., 2009) and to a lesser extent grazing and the occasional harvesting of leaves for fodder. This has created a plant mosaic of different degrees of conservation (Escutia-Lara et al., 2009). Other human disturbances are water extraction for human consumption to fill tank trucks and the use of the rocky shores to wash clothes by the local people in the surrounding communities (Ramíres-Herrejón et al., 2013).
La Mintzita wetland vegetation considered for this study, that comprises the areas that can be classified as fens, is defined by the dominant species Typha domingensis Pers. and Schoenoplectus americanus (Pers.) Volkart ex Schinz & R. Keller, showing a characteristic association in which the former dominates in permanently flooded sites, the latter dominating in sites where the water table is at or a few centimeters bellow the soil surface (Escutia-Lara et al., 2009, Gámez & Lindig-Cisneros, 2014). In addition, in recent years Phragmites australis (Cav.) Trin. ex Steud. has increased its cover in these wetlands, displacing native plants (Escutia-Lara et al., 2012). But the main disturbance factors of concern are fires and to a lesser degree grazing, therefore the purpose of this research project was to analyze the changes in La Mintzita wetland species composition to determine species responses to sustained disturbance by fire and grazing during a ten-year period.
Materials and methods
La Mintzita springs (Fig. 1) are located at an altitude of 1,917 m asl in the municipality of Morelia, state of Michoacán, Mexico, at 101°17’42” W, 19°38’43” N (Inegi, 1998). The region has a temperate sub-humid climate with rains in summer (July to September) and a precipitation to transpiration ratio between 55 and 43.2. Winter rains represent less than 5% of total rainfall. There is little thermal oscillation and a Ganges delta type temperature regime (13 to 34 °C) (García, 1988). The surrounding land vegetation consists mainly of subtropical shrubland, and aquatic plants include submerged vegetation: Nymphaea mexicana Zucc., Stuckenia pectinata (L.) Börner, Fl. Deut. Volk. (syn. Potamogeton pectinatum) and Ceratophyllum demersum L.; floating vegetation is mostly Eichhornia crassipes (Mart.) Solms. The dominant rooted emergent wetland vegetation in this area —the primary focus of this study— are T. domingensis and S. americanus (Rodríguez & Guevara, 2000).
During the decade of study, intentional fires by local inhabitants of the area were started in agricultural lands in February 2006, March 2007, April and July 2009 (these prevented sample taking in this year because the vegetation was destroyed just before the sampling season) and March 2012. These fires covered the total area of study. It was not possible to determine their intensity in terms of maximum temperature reached, almost all of the dry aerial biomass of the wetland plants was consumed by the fires. Salix bonplandiana Kunth was the only species that survived (plants of this species kept their branches and some of their foliage), indicating that the fires were not particularly intense for this species, in terms of maximum temperature and duration. In addition to fires, there was presence of grazing cattle in the wetlands, but in the study area 4 cows and a variable number of horses (from 1 to 4) were present from 2007 to the end of 2013, when they were removed. These animals had a considerable impact since the area was relatively small, giving a density of 1 to 3 animals per hectare, depending on the particular year.
In this study, vegetation sampling was done annually during the rainy season (August-September) over the course of 10 years (2005-2015) within the least disturbed area of the wetlands (Fig. 1). Sampling was done during those months because the aerial parts of almost all species die during the winter, sprout again during spring and reach maturity during summer, when flowering occurs. Five transects, of variable length (30 to 50 m) perpendicular to the water body edge were located 30 m apart from each other. Each transect started at the edge of the emergent hydrophytic vegetation. In each transect, 6 equidistant quadrats measuring 1 m2 were marked. All species present in each quadrat were recorded, cover for each one was assessed by presence/absence in 1 dm² subquadrats, thus allowing for calculating percent cover in the 1 m2 quadrats. Exemplars were collected for identification. Each year the exact same transect route was followed. This method was a modification of the one developed by the “Great Lakes Environmental Indicators Project” a standardized method which allows for efficient sampling of communities and comparison between and among sites (Frieswyk & Zedler, 2006, 2007; Frieswyk et al., 2007). The cover data for each species were averaged for each year and are presented as cover percentages.
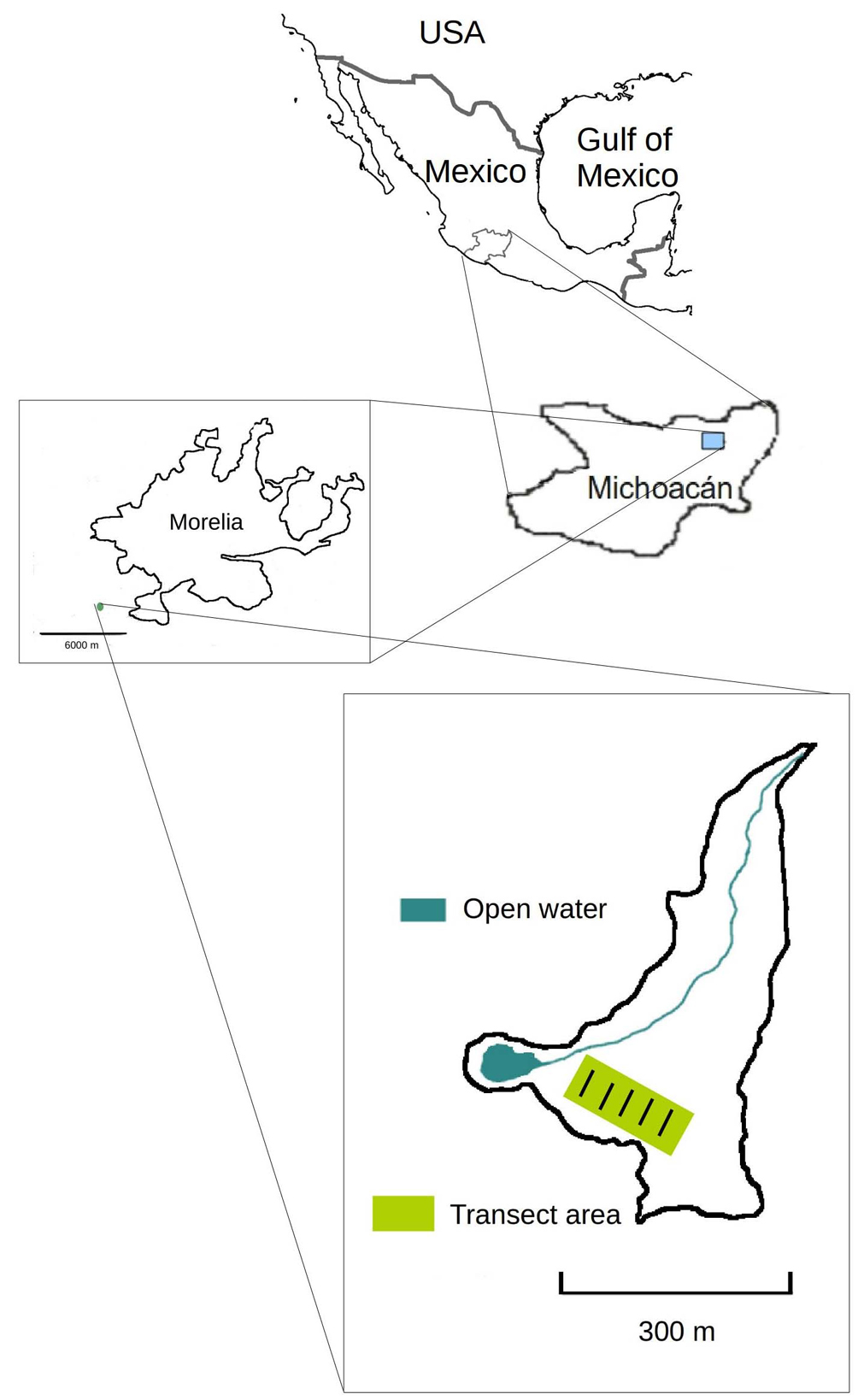
The publications of Calderón-de Rzedowski and Rzedowski (2001, 2004), Lot (2000), Lot and Novelo (2004), Lot et al. (1998, 1999), and Villaseñor and Espinosa (1998) were used for species identification, and the identity of some of the species was corroborated thanks to the collaboration of the University of Wisconsin-Madison (WIS) Herbarium. In addition, the Plants Database (USDA, 2015), which classifies wetland plants as either facultative (that is that occurs in wetlands and non-wetlands) or obligate (that is that almost always occur in wetlands) depending on their occurrence in wetland or non-wetland soils, was consulted to determine the type of habitat of the species found in the area. We corroborated that those species classified as facultative also occurred in non-wetland areas.
Obligate species richness among sampling years was compared with rarefaction curves (sampling effort/estimated species richness) with EstimateS 9.1.0 (Colwell 2013). Cluster analysis was applied to presence-absence data calculating the Jaccard index to compare species composition among years (Manly, 2000) using PAST (Hammer, 2015). Changes in species abundance for the 2 dominant species and 2 indicator species (Carex comosa Boott and Festuca arundinaceae Schreb.) were analyzed with bootstraps, using percent cover as a response variable in each plot by species, with 10,000 resamplings with replacement of the data of the first year and finally comparing means for the first and last year of the study (Crawley, 2007). Carex comosa was chosen as an indicator species because it is an obligate hydrophyte that belongs to a genus that is characteristic of well-preserved wetlands (Werner & Zedler, 2002); Festuca arundinacea was chosen because it is an invasive species of particular management concern because it displaces all natives and is difficult to control (Wasburn & Barnes, 2000). This method allowed us to test if the final mean value corresponded to that expected by random changes. These analyses were performed with R (R Development Core Team, 2008).
Results
During the study period we identified a total of 60 plant species, of which 21 were obligate hydrophytes (35%). The families with the highest number of species were: Poaceae (12), Asteraceae (10) and Cyperaceae (8) (Annex 1). As shown in Fig. 2, the total observed species richness within the transects per year varied between 19 and 41 species, while the number of obligate hydrophytes ranged from 11 to 18, and facultative species from 7 to 23. Obligate species outnumbered facultatives between 2005 and 2014, but in 2015 for the first time facultative species outnumbered obligates.
The difference between years in the expected number of obligate hydrophyte species calculated by rarefaction was not significant, since the comparison of rarefaction curves for the years 2005 and 2015 (the 2 years differing the most) (Fig. 3) had overlapping confidence intervals. For 2005, the computed richness was 15 ± 0.95 species, and it was 18 ± 1.39 species for 2015, hence with a confidence interval of 95%, the minimum value for 2005 was 13.13 species and the maximum for 2015 was 20.73 species. Even though no differences among obligate hydrophyte species richness were found, cluster analysis showed that there is a correlation between fires and community composition changes.
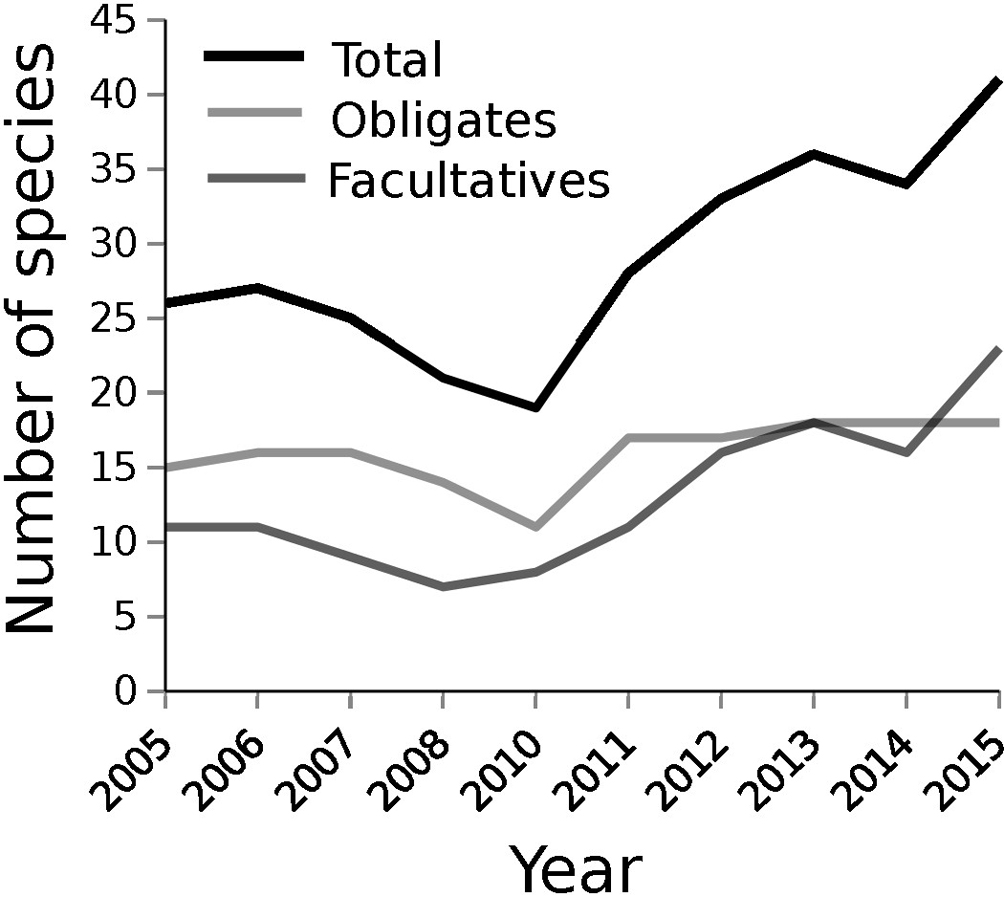
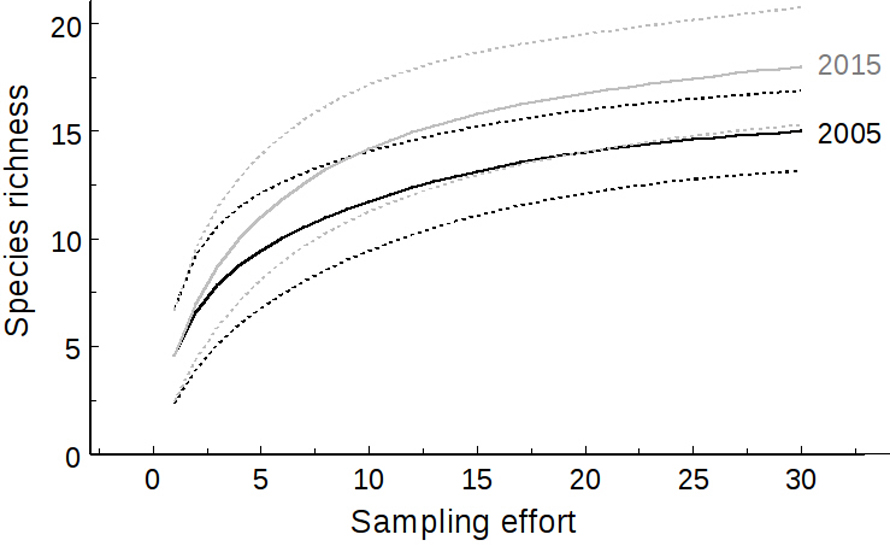
Four large groups form (at 60% similarity). The first group includes the first 4 years, and a major difference in composition between 2005 and the other years of this group that coincides with the first fire that occurred in 2006. Later on, the more intense fires in 2009 that prevented sampling that year brought about another change leaving 2010 in its own group. The third group includes years from 2011 to 2014 when only 1 fire occurred but cattle browsing was constant. After cattle-grazing in the wetlands was banned at the end of 2013, new facultative species became part of the community by 2015, making that year’s species composition different from previous years (Fig. 4). Moreover, changes occurred in the relative abundance (cover percentage) of the most abundant obligate hydrophyte species. The dominant S. americanus and T. domingensis were most abundant in 2008 and 2010, years following those in which the first fires occurred (Fig. 5). In particular S. americanus suffered an initial decline after the first fire that occurred in 2006, causing its cover to drop from 22% in 2006 to 13% in 2007. Bootstrapping showed a significant decline (p < 0.05) in cover for T. domingensis which diminished from 32.79% in 2005 to 27.06% in 2015. In contrast, the other dominant species, S. americanus, registered a significant increase (p < 0.05) in cover from 14.36% in 2005 to 26.39% in 2015, because it was able to colonize areas formerly covered by stands of T. domingensis. The obligate hydrophyte C. comosa showed no significant change (p > 0.05) between the first and last year; nevertheless, its cover gradually increased during the first 4 years and diminished after the 2009 fires. The grass F. arundinacea registered a significant increase (p < 0.05), from 2.49% to 8.06%, especially after cattle grazing in the wetlands was banned. It is important to mention that P. australis, a species that can show invasive behavior, was present only in a few sampling cuadrats and never exceeding 4% cover.
Our data over time from all plots indicates that human disturbances correlate with changes in structure of the wetland vegetation (Fig. 6). Before disturbances were frequent, the vegetation was dominated by a matrix of T. domingensis close to open water and S. americanus in areas when the soil was seasonally inundated. After the years of intermittent fires and grazing, the dominant hydrophytic species (Schoenoplectus and Typha) are now mixed in plots closer to the water body, and facultative species dominate in plots farther away from it, and in particular F. arundinacea is almost monotyphic in sites only seasonally inundated.
Discussion
The changes in vegetation structure between 2005 and 2015 indicated a trend towards a radical change in community composition. The initial species distribution in the wetlands, where S. americanus occupied the areas farthest from the spring lagoon and T. domingensis those closest to it with an intermediate area where both species coexisted, was changed 10 years later as a result of the multiple events of human disturbance, and now a large area is dominated by the introduced grass F. arundinacea, an introduced species of Eurasian origin (Conabio, 2016).
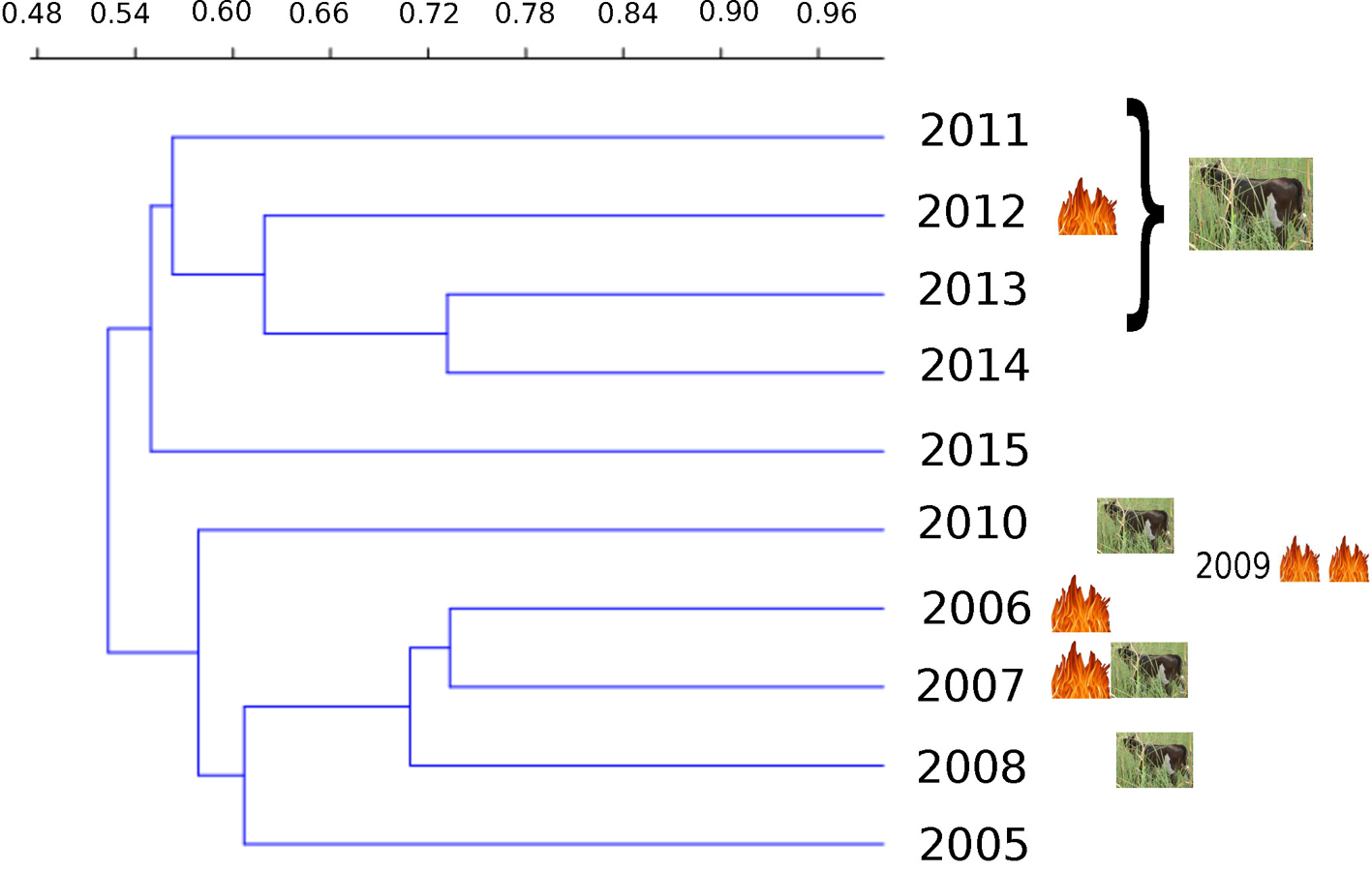
The effect of fire on wetland vegetation depends on the individual responses of the species present. In wetland prairies for example, fire does not have an effect on overall native species composition but can have adverse effects on individual key native species (Clark & Wilson, 2001). In this long term study, fires did not reduce overall obligate hydrophyte species richness, since their numbers remained more or less constant over time, and no local extinctions were detected. Nevertheless, an increase in total species richness occurred due to the incorporation of facultative species capable of tolerating the new site conditions. These trends suggest that the wetland is following a trajectory that might lead to a plant community more similar to upland types. Monitoring obligate hydrophytes for long periods of time is necessary to understand these trajectories and make sound management decisions.
Regarding the dominant species, the cover of T. domingensis was reduced, while it slightly increased in S. americanus so that it occupied areas that had previously been occupied by the former. One important aspect of this trend in the cover of the dominant species is that it could mask changes in the relative cover of the remaining obligate hydrophyte species, making necessary long term monitoring to assess possible effects of altered disturbance regimes.
However, the most noticeable change was that the vast area that was formerly occupied by S. americanus is now dominated by F. arundinacea because, unlike the dominant native species, T. domingensis and S. americanus, which allow the coexistence of native hydrophytes in the community; F. arundinacea gives rise to single-species patches, which could lead to local extinctions if the area covered by this species continues to expand.
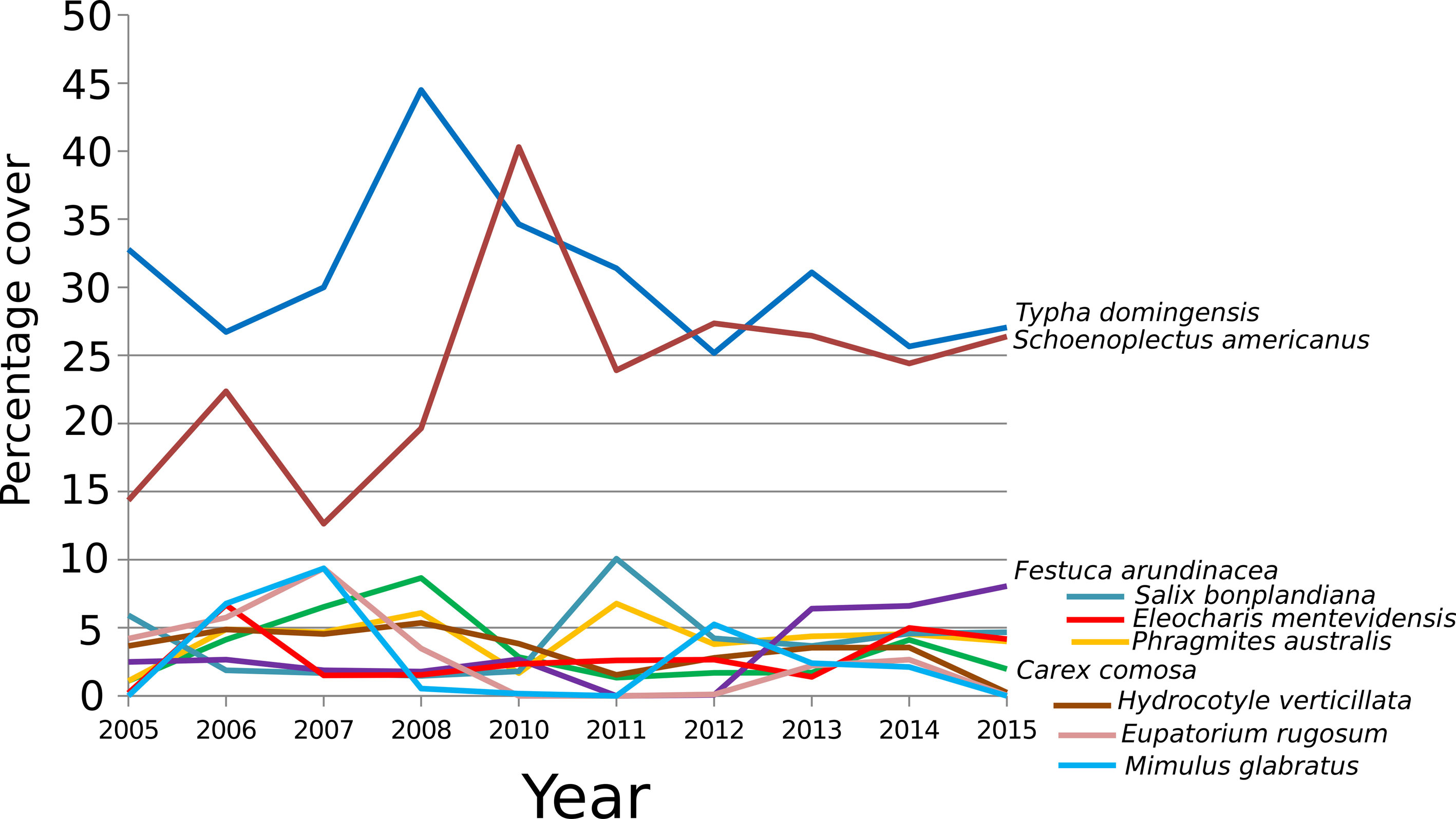
We observed a temporal correlation between intentional fires on the wetlands and the changes in plant composition. This coincides with previous studies that show how fires allow the colonization by opportunistic species (Bisson et al., 2003), and also that there can be important spatial changes in vegetation cover types in wetlands (Smith III et al., 2013). After the first fire which occurred in February 2006, when plants were dormant, the plant community, showed a 60% resemblance to the initial one, and this situation remained the same until 2008. The 2009 fires, that occurred when the plants were sprouting in April and again in July, caused the wetlands considerable damage and brought about a drastic change in their composition. The following year the resemblance to the original composition was 57% but by 2011 it fell to 52%, indicating non-immediate effects. After these fires, the composition was radically altered as shown by the 2 groups that resulted when applying cluster analysis, one from 2005 to 2010 and the other from 2011 to 2015. In the latter group, the composition for 2015 was remarkably different from that of previous years, as a consequence of the growing abundance of F. arundinacea, which was to a great extent due to the ban on cattle grazing on the wetlands. But most certainly, the most radical change suffered by La Mintzita vegetation was due mainly to the intense and consecutive fires that occurred in 2009. Moreover, the first one occurred at the end of the dry season when the plants were just beginning to sprout, while the second one prevented their development at the end of their growth period thus also preventing the accumulation of reserves, in particular that of starch in rhizomes.
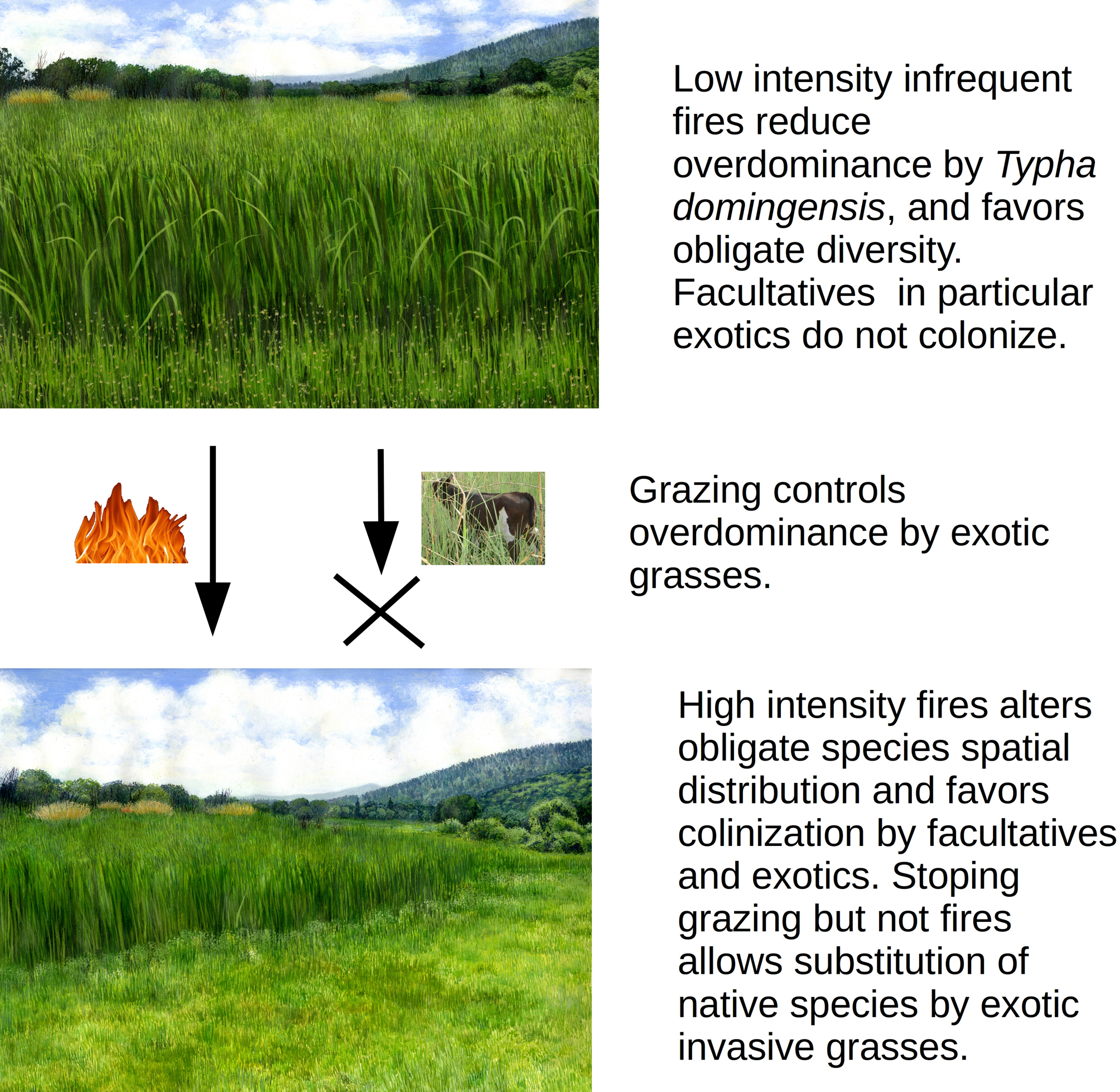
In a previous short term study of the same area before the major fires of 2009 (Escutia-Lara et al., 2009), we concluded that fires might play a positive role in maintaining plant diversity in Western Mexico wetlands. This can still be the case for low-intensity infrequent fires, that open the canopy of the obligate dominant hypdrophytes (Typha domingensis and S. americanus), and allow other natives to be present as shown by harvesting experiments in the same wetlands (Hall et al., 2008) and reducing its competitiveness (Montejo-Mayo et al., 2015). But intense and frequent fires, especially during the growing season, alter significantly the wetland plant community composition. Also, cattle browsing interacts with other disturbances in still not well understood ways. Here, we have shown that removing the cattle was correlated with an increment in the abundance of the invasive F. arundinacea, and that these areas will probably require active management for restoration.
Human disturbances can have radical and devastating effects on ecosystem structure and function. In wetlands, human disturbance can cause devastating effects and environmental degradation (Chokkalingam & Suyanto, 2004). Long term studies are necessary to understand trends and trajectories, which will help recommend alternative management practices.
Appendix. Species present throughout the years of study, if they are obligate or facultative wetland species, and the percentage of coverage.
Appendix. Continued… |
||||||||||||
Species |
Family |
Habitat |
2005 |
2006 |
2007 |
2008 |
2010 |
2011 |
2012 |
2013 |
2014 |
2015 |
Species |
Family |
Habitat |
2005 |
2006 |
2007 |
2008 |
2010 |
2011 |
2012 |
2013 |
2014 |
2015 |
Acacia farnesiana (L.) Willd. |
Fabaceae |
facultative |
0.00 |
0.00 |
0.00 |
0.00 |
0.00 |
0.00 |
0.00 |
0.00 |
0.00 |
0.10 |
Arenaria paludicola B.L. Robins |
Caryophyllaceae |
obligate |
2.17 |
0.34 |
0.87 |
0.47 |
0.00 |
0.53 |
0.27 |
0.33 |
1.52 |
0.73 |
Asclepias curassavica L. |
Asclepiadaceae |
facultative |
0.00 |
0.00 |
0.00 |
0.00 |
0.00 |
0.17 |
0.00 |
0.00 |
0.00 |
0.00 |
Berula erecta (Huds.) Couille |
Apiaceae |
obligate |
2.85 |
3.43 |
0.37 |
0.07 |
0.33 |
2.70 |
1.90 |
2.96 |
2.72 |
3.80 |
Bidens aurea (Ait.) Sherff |
Asteraceae |
facultative |
0.54 |
0.00 |
0.00 |
0.00 |
0.00 |
0.00 |
0.33 |
0.00 |
0.00 |
0.13 |
Bidens cernua L. |
Asteraceae |
obligate |
0.95 |
0.00 |
0.67 |
0.00 |
0.00 |
0.00 |
0.00 |
0.00 |
0.00 |
0.00 |
Carex comosa Boott |
Cyperaceae |
obligate |
1.08 |
4.15 |
6.53 |
8.66 |
2.83 |
1.33 |
1.70 |
1.70 |
4.11 |
1.97 |
Carex longii Mack. |
Cyperaceae |
facultative |
0.00 |
0.00 |
0.00 |
0.00 |
0.00 |
0.00 |
0.00 |
0.00 |
0.00 |
0.03 |
Commelina diffusa Burm. f. |
Commelinaceae |
facultative |
0.00 |
0.00 |
0.00 |
0.00 |
0.00 |
0.10 |
0.00 |
0.00 |
0.00 |
0.00 |
Cosmos bipinnatus Cav. |
Asteraceae |
facultative |
0.00 |
0.00 |
0.00 |
0.00 |
0.00 |
0.67 |
1.67 |
0.00 |
0.00 |
0.83 |
Cosmos sulphureus Cav. |
Asteraceae |
facultative |
0.00 |
0.00 |
0.00 |
0.00 |
0.00 |
0.00 |
0.00 |
0.37 |
0.13 |
0.03 |
Cyperus eragrostis Lam. |
Cyperaceae |
facultative |
0.14 |
0.00 |
0.00 |
0.00 |
0.10 |
0.07 |
0.93 |
0.00 |
0.33 |
0.33 |
Cyperus niger R. y P. |
Cyperaceae |
facultative |
0.14 |
0.62 |
0.00 |
0.00 |
2.23 |
0.23 |
0.57 |
0.20 |
0.30 |
0.67 |
Distichlis spicata (L.) Greene. |
Poaceae |
facultative |
0.00 |
0.00 |
0.00 |
0.00 |
0.00 |
0.00 |
0.00 |
0.00 |
0.00 |
0.67 |
Eichhornia crassipes (C. Martius) Sol Ms-Laub. |
Pontederiaceae |
obligate |
0.68 |
0.00 |
0.00 |
0.00 |
0.00 |
0.00 |
0.00 |
0.13 |
0.07 |
1.17 |
Eleocharis densa Benth. |
Cyperaceae |
obligate |
0.00 |
0.00 |
0.00 |
0.00 |
0.00 |
0.00 |
0.00 |
0.00 |
0.00 |
5.43 |
Eleocharis montevidensis Kunth |
Cyperaceae |
facultative |
0.19 |
6.72 |
1.50 |
1.54 |
2.33 |
2.60 |
2.67 |
1.40 |
4.97 |
4.17 |
Eleusine indica (L.) Gaertn. |
Poaceae |
facultative |
0.00 |
0.00 |
0.00 |
0.00 |
0.00 |
0.00 |
0.00 |
0.13 |
0.00 |
0.03 |
Epilobium ciliatum Raf. |
Onagraceae |
facultative |
0.95 |
0.00 |
0.00 |
0.00 |
0.13 |
0.00 |
0.00 |
0.00 |
0.00 |
0.00 |
Erigeron scaberrimus Gardner |
Asteraceae |
facultative |
0.00 |
0.00 |
0.00 |
0.00 |
0.00 |
0.73 |
0.00 |
0.00 |
0.33 |
0.07 |
Eupatorium rugosum Houtt. |
Asteraceae |
facultative |
4.20 |
5.80 |
9.37 |
3.48 |
0.00 |
0.00 |
0.10 |
2.20 |
2.65 |
0.03 |
Festuca arundinacea Schreb. |
Poaceae |
facultative |
2.49 |
2.68 |
1.87 |
1.77 |
2.67 |
0.00 |
0.07 |
6.39 |
6.60 |
8.07 |
Fraxinus uhdei (Wenz.) Lingelsh. |
Oleaceae |
facultative |
0.00 |
0.00 |
0.00 |
0.00 |
0.00 |
0.17 |
0.00 |
0.60 |
0.00 |
0.03 |
Galium trifidium L. |
Rubiaceae |
facultative |
0.00 |
0.62 |
0.33 |
0.13 |
0.17 |
0.20 |
0.30 |
1.90 |
1.26 |
0.27 |
Gnaphalium americanum (P. Mill.) Wedell |
Asteraceae |
facultative |
0.00 |
0.14 |
1.33 |
0.00 |
0.00 |
0.00 |
0.00 |
0.37 |
0.00 |
0.00 |
Hydrocotyle ranunculoides L. f. |
Apiaceae |
obligate |
0.00 |
0.27 |
0.07 |
0.00 |
0.00 |
0.00 |
0.73 |
2.53 |
0.40 |
0.00 |
Hydrocotyle verticillata Thunb. |
Apiaceae |
obligate |
3.66 |
4.91 |
4.53 |
5.35 |
3.83 |
1.53 |
2.80 |
3.53 |
3.55 |
0.23 |
Lemna gibba L. |
Lemnaceae |
obligate |
2.17 |
0.41 |
0.33 |
0.00 |
0.00 |
0.47 |
0.10 |
0.00 |
0.66 |
2.07 |
Lepidium virginicum L. |
Brassicaceae |
facultative |
0.00 |
0.00 |
0.00 |
0.00 |
0.00 |
0.00 |
0.00 |
0.10 |
0.00 |
0.00 |
Lobelia laxiflora Kunth |
Campanulaceae |
facultative |
0.27 |
0.17 |
0.00 |
0.00 |
0.00 |
0.00 |
0.00 |
0.00 |
0.00 |
0.00 |
Lythrum vulneraria L. |
Lythraceae |
obligate |
1.22 |
0.48 |
2.33 |
3.51 |
0.07 |
0.33 |
3.77 |
2.10 |
1.19 |
1.20 |
Melinis repens (Willd.) Zizka |
Poaceae |
facultative |
0.00 |
0.00 |
0.00 |
0.00 |
0.00 |
0.00 |
0.33 |
0.00 |
0.00 |
1.73 |
Mimulus glabratus Kunth |
Scrophulariaceae |
obligate |
0.00 |
6.83 |
9.37 |
0.54 |
0.17 |
0.00 |
5.27 |
2.40 |
2.12 |
0.00 |
Oenothera rosea L´Hér. ex Ait. |
Onagraceae |
facultative |
0.00 |
0.00 |
0.00 |
0.00 |
0.00 |
0.00 |
0.00 |
0.13 |
0.00 |
0.00 |
Panicum hallii Vasey |
Poaceae |
facultative |
1.36 |
0.14 |
0.17 |
0.13 |
0.00 |
0.10 |
1.50 |
0.10 |
0.10 |
0.40 |
Panicum sucosum Hitch. and Chase |
Poaceae |
facultative |
0.00 |
0.51 |
0.00 |
0.00 |
0.00 |
0.00 |
0.00 |
0.00 |
0.00 |
0.00 |
Passiflora subpeltata Ortega |
Passifloraceae |
facultative |
0.00 |
0.00 |
0.00 |
0.00 |
0.00 |
0.00 |
0.00 |
0.00 |
0.00 |
0.07 |
Phragmites australis (Cav.) Trin. ex Steud. ( ITIS ). |
Poaceae |
obligate |
1.08 |
4.91 |
4.67 |
6.09 |
1.67 |
6.77 |
3.80 |
4.36 |
4.54 |
4.00 |
Plantago major L. |
Plantaginaceae |
facultative |
0.00 |
0.00 |
0.00 |
0.00 |
0.00 |
0.00 |
0.00 |
0.00 |
0.00 |
0.07 |
Poaceae sp. |
Poaceae |
facultative |
2.52 |
0.99 |
0.00 |
1.24 |
1.60 |
0.00 |
0.00 |
0.00 |
0.10 |
0.00 |
Polygonum hydropiperoides Michx. |
Polygonaceae |
0.14 |
1.20 |
1.67 |
0.30 |
1.40 |
4.60 |
4.90 |
1.73 |
2.72 |
0.50 |
|
Polygonum lapathifolium L. |
Polygonaceae |
0.41 |
1.51 |
5.73 |
0.40 |
0.00 |
1.07 |
3.13 |
0.50 |
1.13 |
0.37 |
|
Polypogon interruptus Kunth |
Poaceae |
0.00 |
1.17 |
1.20 |
0.00 |
3.33 |
6.03 |
0.87 |
0.20 |
0.17 |
0.17 |
|
Polypogon monspeliensis (L.) Desf. |
Poaceae |
0.00 |
0.00 |
1.00 |
0.00 |
0.00 |
0.67 |
0.17 |
0.27 |
0.00 |
0.03 |
|
Psilactis asteroides A.Gray |
Asteraceae |
0.00 |
0.00 |
0.00 |
0.00 |
0.00 |
0.00 |
2.97 |
0.00 |
0.00 |
0.00 |
|
Rorippa palustris (L.) Besser |
Brassicaceae |
0.00 |
0.10 |
0.00 |
0.10 |
0.00 |
0.07 |
0.10 |
0.07 |
0.17 |
0.00 |
|
Rubus fruticosus L. |
Rosaceae |
0.00 |
0.00 |
0.00 |
0.00 |
0.00 |
0.00 |
0.00 |
0.33 |
0.27 |
0.03 |
|
Sagittaria latifolia Willd. |
Alismataceae |
0.00 |
0.00 |
0.00 |
0.00 |
0.23 |
0.50 |
1.00 |
0.93 |
0.50 |
0.57 |
|
Salix bonplandiana Kunth |
Salicaceae |
5.91 |
1.89 |
1.67 |
1.47 |
1.80 |
10.07 |
4.23 |
3.66 |
4.57 |
4.67 |
|
Schoenoplectus americanus (Pers.) Volkart ex Schinz and Keller |
Cyperaceae |
14.36 |
22.54 |
12.63 |
19.63 |
40.30 |
23.90 |
27.50 |
26.44 |
24.40 |
26.40 |
|
Schoenoplectus tabernaemontani (C.C. Gmeling) Palla |
Cyperaceae |
1.36 |
0.55 |
0.50 |
0.10 |
0.17 |
0.60 |
0.20 |
0.17 |
0.33 |
0.20 |
|
Setaria parviflora (Poir.) M.Kerguelen |
Poaceae |
0.00 |
0.00 |
0.00 |
0.00 |
0.00 |
0.00 |
0.00 |
0.00 |
0.00 |
0.23 |
|
Sida rhombifolia L. |
Malvaceae |
0.00 |
0.00 |
0.00 |
0.00 |
0.00 |
2.40 |
0.00 |
0.33 |
0.17 |
0.83 |
|
Solanum americanum P. Mill. |
Solanaceae |
0.14 |
0.00 |
1.30 |
0.54 |
0.00 |
0.00 |
0.00 |
0.07 |
0.07 |
0.03 |
|
Solidago velutina DC. |
Asteraceae |
0.00 |
0.00 |
0.00 |
0.00 |
0.00 |
0.00 |
0.00 |
0.03 |
0.03 |
0.00 |
|
Sonchus asper (L.) Hill. |
Asteraceae |
0.00 |
0.00 |
0.00 |
0.00 |
0.00 |
0.00 |
0.37 |
0.20 |
0.07 |
0.00 |
|
Spirodela polyrhiza (L.) Schleid |
Lemnaceae |
0.00 |
0.00 |
0.00 |
0.00 |
0.00 |
0.00 |
0.00 |
0.00 |
0.00 |
0.03 |
|
Typha domingensis Presl. |
Typhaceae |
32.79 |
26.93 |
30.00 |
44.48 |
34.63 |
31.40 |
25.30 |
31.10 |
25.65 |
27.07 |
|
Tripoganda purpurascens (Schauer) Handlos. |
Commelinidae |
0.00 |
0.00 |
0.00 |
0.00 |
0.00 |
0.00 |
0.10 |
0.00 |
0.00 |
0.00 |
|
Urochloa maxima (Jacq.) R. Webster |
Poaceae |
0.00 |
0.00 |
0.00 |
0.00 |
0.00 |
0.00 |
0.37 |
0.00 |
2.12 |
0.10 |
|
Verbena carolina L. |
Verbenaceae |
0.00 |
0.00 |
0.00 |
0.00 |
0.00 |
0.00 |
0.00 |
0.00 |
0.00 |
0.43 |
References
Baruch, Z., & Goldstein, G. (1999). Leaf construction, nutrient concentration, and net CO2 assimilation of native and invasive species in Hawaii. Oecologia, 121, 183–192.
Bisson, P. A., Rieman, B. E., Luce, C., Hessburg, P. F., Lee, D. C., Kershner, J. F. et al. (2003). Fire and aquatic ecosystems of the western USA: current knowledge and key questions. Forest Ecology and Management, 178, 213–229.
Blom, C. W. P. M., & Voesenek, L. A. C. J. (1996). Flooding: the survival strategies of plants. TREE, 11, 290–295.
Bond, W. J., & Keleey, J. E. (2005). Fire as a global ‘herbivore’: the ecology and evolution of flammable ecosystems. Trends in Ecology and Evolution, 20, 387–394.
Brun, A., Campanella, O., Oggero, A., & Suárez, S. (2012). Cambios en el IVN y su relación con disturbios antrópicos en la vegetación serrana de Córdoba, Argentina. Revista de Teledetección, 37, 34–41.
Calderón-de Rzedowski, G., & Rzedowski, J. (2001). Flora fanerogámica del Valle de México. Comisión Nacional para el Estudio de la Biodiversidad/ México D.F.: Instituto de Ecología, A.C.
Calderón-de Rzedowski, G., & Rzedowski, J. (2004). Manual de malezas de la región de Salvatierra, Guanajuato. Flora del Bajío y regiones adyacentes. Pátzcuaro, Michoacán, México: Instituto de Ecología, A.C.
Carter, M. F., & Grace, J. B. (1990). Relationships flooding tolerance, life history, and short term competitive performance in three species of Polygonum. American Journal of Botany, 77, 381–387.
Chokkalingam, U., & Suyanto, S. (2004). Fire, livelihoods and environmental degradation in the wetlands of Indonesia: a vicious cycle (No. CIFOR Fire Brief no. 3, p. 4p). Bogor, Indonesia: CIFOR.
Clark, D. L., & Wilson, M. V. (2001). Fire, mowing, and hand-removal of woody species in restoring a native wetland prairie in the Willamette Valley of Oregon. Wetlands, 21, 135–144.
Colwell, R. K. (2013). EstimateS: statistical estimation of species richness and shared species from samples, version 9.1.0. Retrieved on August 2nd 2018, from: http://viceroy.eeb.uconn.edu/estimates/
Conabio (Comisión Nacional para el Estudio de la Biodiversidad). (2016). Festuca arundinacea. Retrieved on August 20th, from: http://www.conabio.gob.mx/malezasdemexico/poaceae/festuca-arundinacea/fichas/ficha.htm
Contini, C., & Cannicci, S. (2009). Management of grazing in wetlands. In Biodiversity, conservation and habitat management – Volume 1 (pp. 302–325). Encyclopedia of Life Support Systems (EOLSS). Oxford. United Kingdom.
Crawley, M. J. (2007). The R book. London: John Wiley and Sons.
Cronk, J. K. & Fennessy, M. S. (2001). Wetland plants: biology and ecology. Boca Raton: CRC Press/ Lewis Publishers.
Dwire, K. A., & Boone-Kauffman, J. B. (2003). Fire and riparian ecosystems in landscapes of the western USA. Forest Ecology and Management, 178, 61–74.
Escutia-Lara, Y., Lara-Cabrera, S., Gómez-Romero, M., & Lindig-Cisneros, R. (2012). Common reed (Phragmites australis) harvest as a control method in a Neotropical wetland in Western México. Hydrobiológica, 22, 125–131.
Escutia-Lara, Y., Lara-Cabrera, S., & Lindig-Cisneros, R. (2009). Efecto del fuego y dinámica de las hidrófitas emergentes en el humedal de La Mintzita, Michoacán, México. Revista Mexicana de Biodiversidad, 80, 771–778.
Frieswyk, C. B., Johnston, C., & Zedler, J. B. (2007). Quantifying and qualifying dominance in vegetation. Journal of Great Lakes Research, 33, 125–135.
Frieswyk, C. B., & Zedler, J. B. (2006). Do seed banks confer resilience to coastal wetlands invaded by Typha x glauca? Canadian Journal of Botany, 84, 1882–1893.
Frieswyk, C. B., & Zedler, J. B. (2007). Vegetation change in Great Lakes coastal wetlands: Deviation from the historical cycle. Journal of Great Lakes Research, 33, 366–380.
Gabrey, S. W., & Afton, A. D. (1999). Effects of winter burning and structural marsh management on vegetation and winter bird abundance in the Gulf Coast Chenier Plain. Wetlands, 19, 593–606.
Gabrey, S. W., & Afton, A. D. (2001). Plant community composition and biomass in Gulf Coast Chenier Plain marshes: responses to winter burning and structural marsh management. Environmental Management, 27, 281–293.
Gámez, S., & Lindig-Cisneros, R. (2014). Efecto de la profundidad del agua en la germinación de Typha domingensis. Biológicas Revista de la DES Ciencias Biológico Agropecuarias Universidad Michoacana de San Nicolás de Hidalgo, 15, 14–18.
García, E. (1988). Modificaciones al sistema de clasificación climática de Copen, 4ª edición. México D.F.: CETENAL.
Hall, S., Zedler J. B., & Lindig-Cisneros, R. (2008). Does harvesting sustain diversity in Central Mexican Wetlands? Wetlands, 28, 776–792.
Hammer, O. (2015). Programa estadístico Past, versión 3.8. Disponible en: http://folk.uio.no/ohammer/past/
Hernández, E. C., Reiss, K. C., & Brown, M. T. (2015). Effect of time on consistent and repeatable macrophyte index for wetland condition. Ecological Indicators, 52, 558–566.
Hochkirchi, A., & Adorf, F. (2007). Effects of prescribed burning and wildfires on Orthoptera in Central European peat bogs. Environmental Conservation, 34, 225–235.
Inegi (Instituto Nacional de Estadística y Geografía). (1998). “Carta Topográfica” Morelia, E14-1. Escala 1:250,000. Michoacán, México.
Johnson, S. R., & Knapp, A. K. (1993). The effect of fire on gas exchange and aboveground biomass production in annually vs. biennially burned Spartina pectinata wetlands. Wetlands, 13, 299–303.
Keddy, P. A. (2010). Wetland ecology: principles and conservation. Cambridge: Cambridge University Press.
Kost, M. A., & Steven, D. (2001). Plant community responses to prescribed burning in Wisconsin sedge meadows. Natural Areas Journal, 687, 36–45.
Laubhan, M. K. (1995). Effects of prescribed fire on moist soil vegetation and soil macronutrients. Wetlands, 15, 159–166.
Lot, A. (2000). Plantas acuáticas en los jardines botánicos: ideas sobre el desarrollo de la colecciones. Boletín Amaranto, 13, 13–25.
Lot, A., & Novelo, A. (2004). Iconografía y estudio de plantas acuáticas de la ciudad de México y sus alrededores. Méxioco, D.F.: Instituto de Biología, Dirección de Divulgación Científica, UNAM.
Lot, A., Novelo, A., Olvera, M., & Ramírez, P. (1999). Catálogo de Angiospermas acuáticas de México. Hidrófitas estrictas emergentes, sumergidas y flotantes. Cuadernos del Instituto de Biología, UNAM. Núm.33. México D.F.: UNAM.
Lot, A., Novelo, A., & Ramírez-García, P. (1998). Diversidad de la flora acuática mexicana. In T. P. Ramamoorthy, R. Bye, A. Lot y J. Fa (Eds.), Diversidad biológica de México: orígenes y distribución. Instituto de Biología UNAM. México. (pp. 563–580).
Manly, B. F. J. (2000). Multivariate statistical methods: a primer, 2a Edition. London: Chapman and Hall/ CRC.
Miller, S. J. (1997). The use of fire in wetland preservation and restoration: are there risks? In T. L. Pruden, & L. A. Brennan (Eds.), Fire in ecosystem management: shifting the paradigm from suppression to prescription, 20th Ed. (pp. 127–139). Tallahassee, FL: Tall Timbers Research Station.
Montejo-Mayo, W., del Val, E., Gómez-Romero, M., de la Barrera, E., & Lindig-Cisneros, R. (2015). Interactions between dominant hydrophytic species of the wetlands of western Mexico mediated by fire and nitrate concentration. Polibotánica, 40, 153–161.
Morris, K., & Reich, P. (2013). Understanding the relationship between grazing and wetland condition. Arthur Rylah Institute for Environmental Research Technical Report Series No. 252. Heidelberg, Victoria: Department of Environment and Primary Industry.
Pettit, N. E., & Naiman, R. J. (2007). Fire in the riparian zone: characteristics and ecological consequences. Ecosystems, 10, 673–687.
R Development Core Team. (2008). R: a language and environment for statistical computing. R Foundation for Statistical Computing, Vienna, Austria. Retrieved on January 12th 2008 from: http://www.R-project.org
Ramírez-Herrejón, J. P. R., Nava, M. M., Tinoco, C. I. S., & Zubieta, T. L. (2013). Algunos aspectos reproductivos de Zoogoneticus quitzeoensis; Hubbs and Turner (1939) (Osteichtyes-Goodeidae) en la represa La Mintzita Morelia, Michoacán, México. Biológicas Revista de la DES Ciencias Biológico Agropecuarias Universidad Michoacana de San Nicolás de Hidalgo, 9, 63–71.
Rodríguez, J., & Guevara, F. (2000). Angiospermas: catálogo de la biodiversidad en Michoacán. Ciudad de México: SEDUE.
Sah, J. P., Ross, M., Saha, M. S., Minchin, P., & Sadle, J. (2014). Trajectories of vegetation response to water management in Taylor Slough, Everglades National Park, Florida. Wetlands, 34, 65–79.
Sand-Jensen, K., & Frost-Christensen, H. (1999). Plant growth and photosynthesis in the transition zone between land and stream. Aquatic Botany, 63, 23–35.
Smith III, T. J., Foster, A. M., Tiling-Range, G., & Jones, J. W. (2013). Dynamics of mangrove–marsh ecotones in subtropical coastal wetlands: fire, sea-level rise, and water levels. Fire Ecology, 9, 66–77.
USDA (United States Department of Agriculture). (2015). Plants database. Retrieved on August 16, 2015 from: http://plants.usda.gov/index.html
Villaseñor, J. L., & Espinosa-García, F. J. (1998). Catálogo de malezas de México. Mexico City: Universidad Nacional Autónoma de México/ Fondo de Cultura Económica.
Washburn, B. E., & Barnes, T. G. (2000). Postemergence tall fescue (Festuca arundinacea) control at different growth stages with glyphosate and AC 263,222. Weed Technology, 14, 223–230.
Werner, K. J., & Zedler, J. B. (2002). How sedge meadow soils, microtopography, and vegetation respond to sedimentation. Wetlands, 22, 451–466.
Wu, Y., Rutchey, K., Newman, S., Miao, S., Wang, N., Sklar, F. H. et al. (2012). Impacts of fire and phosphorus on sawgrass and cattails in an altered landscape of the Florida Everglades. Ecological Processes, 1, 3–11.